Dr Isabella Guido
About
Biography
Isabella Guido is a Senior Lecturer in Experimental Soft Matter Physics at the University of Surrey.
She received her PhD at TU Berlin (Berlin Institute of Technology) in Germany in 2010 with her thesis on dielectrophoretic effects of electric fields on mammalian cells. After her PhD, she moved to the Peking University, China, as postdoctoral researcher, where she worked on cell mechanics and microfluidics for cellular electroporation. In 2013, she moved to Göttingen, Germany, to the Max Planck Institute for Dynamics and Self-Organization, where she worked as a research associate on cellular migration in electric fields. IIn 2015, she expanded her research interests towards synthetic biology and became group leader of the Synthetic Active Systems group. In 2022, she was appointed senior lecturer in physics at the University of Surrey.
Areas of specialism
ResearchResearch interests
My research interests lie at the intersection of active matter physics and synthetic biology. With this interdisciplinary work we aim to develop minimal synthetic structures that are able to mimic natural systems, using a few biological building blocks such as microtubules and motor proteins. They represent innovative model systems for the investigation of emergent principles that govern the dynamic organization of living matter.
One of our recent projects involved the development of minimal beating systems, resembling cilia/flagella. These systems consist of a few microtubules and motor proteins. Our goal is to uncover the mechanism underlying the beating pattern of cilia and their hydrodynamic coupling for fluid transport.
We are also investigating 3D active nematics and active biopolymer network to understand the relevance of the biopolymer orientational order in biological context and the influence of the geometrical enviroment they act in. Active nematics are an attractive topic in the field of active matter and the extension to 3D systems offers many possibilities for fascinating new physical phenomena in soft matter.
Currently, my research focus is on understanding the mechanism leading to cellular rotational symmetry breaking by using a synthetic biomimetic cell-like system.
Join my group and share with us this exciting scientific journey!
Research interests
My research interests lie at the intersection of active matter physics and synthetic biology. With this interdisciplinary work we aim to develop minimal synthetic structures that are able to mimic natural systems, using a few biological building blocks such as microtubules and motor proteins. They represent innovative model systems for the investigation of emergent principles that govern the dynamic organization of living matter.
One of our recent projects involved the development of minimal beating systems, resembling cilia/flagella. These systems consist of a few microtubules and motor proteins. Our goal is to uncover the mechanism underlying the beating pattern of cilia and their hydrodynamic coupling for fluid transport.
We are also investigating 3D active nematics and active biopolymer network to understand the relevance of the biopolymer orientational order in biological context and the influence of the geometrical enviroment they act in. Active nematics are an attractive topic in the field of active matter and the extension to 3D systems offers many possibilities for fascinating new physical phenomena in soft matter.
Currently, my research focus is on understanding the mechanism leading to cellular rotational symmetry breaking by using a synthetic biomimetic cell-like system.
Join my group and share with us this exciting scientific journey!
Sustainable development goals
My research interests are related to the following:
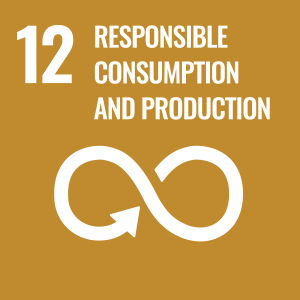
Publications
Living cells have the ability to detect electric fields and respond to them with directed migratory movements. Many proteomic approaches have been adopted in the past to identify the molecular mechanism behind this cellular phenomenon. However, how the cells sense the electric stimulus and transduce it into directed cell migration is still under discussion. Many eukaryotic cells react to applied electric stimulation, including Dictyostelium discoideum cells. We use them as model system for studying cell migration in electric fields, also known as electrotaxis. Here we report the protocols that we developed for our experiments. Our experimental outcomes helped us to characterize: (i) the memory that cells have in a varying electric field, which we defined as temporal electric persistence; and (ii) the accelerating motion of cells along their paths over the electric exposure time. We also report on the analysis of the role that conditioned medium factor (CMF), a protein secreted by cells when they begin to starve, plays in the mechanism of electric sensing. The results of this study can contribute to the understanding of the electrical sensing of cells and its transduction into directed cell migration.
Active networks made of biopolymers and motor proteins are valuable bioinspired systems that have been used in the last decades to study the cytoskeleton and its self-organization under mechanical stimulation. Different techniques are available to apply external mechanical cues to such structures. However, they often require setups that hardly mimic the biological environment. In our study we use an evaporating sessile multi-component droplet to confine and mechanically stimulate our active network made of microtubules and kinesin motor proteins. Due to the well-characterized flow field inside an evaporating droplet, we can fathom the coupling of the intrinsic activity of the biological material with the shear stress generated by the flow inside the droplet. We observe the emergence of a dynamic pattern due to this combination of forces that vary during the evaporation period. We delineate the role that the composition of the aqueous environment and the nature of the substrate play in pattern formation. We demonstrate that evaporating droplets may serve as bioreactors that supports cellular processes and allows investigation on the dynamics of membraneless compartments. Such a setup is an original tool for biological structures to understand the mechanisms underlying the activity of the cytoskeleton under stress and, on the other hand, to investigate the potential of such adaptive materials compared to conventional materials.
Metal-induced energy transfer (MIET) imaging is an easy-to-implement super-resolution modality that achieves nanometer resolution along the optical axis of a microscope. Although its capability in numerous biological and biophysical studies has been demonstrated, its implementation for live-cell imaging with fluorescent proteins is still lacking. Here, we present its applicability and capabilities for live-cell imaging with fluorescent proteins in diverse cell types (adult human stem cells, human osteo-sarcoma cells, and Dictyostelium discoideum cells), and with various fluorescent proteins (GFP, mScarlet, RFP, YPet). We show that MIET imaging achieves nanometer axial mapping of living cellular and subcellular components across multiple time scales, from a few milliseconds to hours, with negligible phototoxic effects.
The propulsion of motile cells such as sperms and the transport of fluids on cell surfaces rely on oscillatory bending of cellular appendages that can perform periodic oscillations. These structures are flagella and cilia. Their beating is driven by the interaction between microtubules and motor proteins and the mechanism regulating this is still a puzzle. One approach to address this issue is the assembling of synthetic minimal systems by using natural building blocks, e.g., microtubules and kinesin motors, which undergo persistent oscillation in the presence of ATP. An example of an autonomous molecular system is reported in this chapter. It dynamically self-organizes through its elasticity and the interaction with the environment represented by the active forces exerted by motor proteins. The resulting motion resembles the beating of sperm flagella. Assembling such minimal systems able to mimic the behavior of complex biological structures might help to unveil basic mechanisms underlying the beating of natural cilia and flagella.
The cellular mechanical properties and changes in cell mechanics are of enormous relevance in many cellular processes and play an important role in the normal or pathological development of cells. We have developed a new technique, the dielectrophoretic stretcher, for analyzing cellular mechanical behavior by stretching cells between microelectrodes through dielectrophoresis. We obtained reliable data that allowed us to unambiguously distinguish between two cell types, one of cancerous origin (MCF-7) and the other from related non-cancerous tissue (MCF-10A). In a second step, this novel technique was integrated into a simple microsystem and was tested by stretching murine cells (Jurkat). These tests verified the compatibility of the dielectrophoretic stretcher with modern microfluidic environments. Our system can additionally be combined with dielectrophoretic manipulation units for aligning, sorting, or positioning cells. It has the potential for automation and, thus, for achieving high throughput.
The stiffness of cells is strongly influenced by specifics in their cytoskeleton. Their mechanical properties, therefore, are an easily accessible parameter for distinguishing between cells. Monitoring the cell deformation under the influence of external forces provides all the advantages of a label-free characterization, e.g. avoiding interference with the physiological state and obviating time-consuming labeling steps that also increase the intricacy of microsystems. In this way, individual cells can be identified in on-chip microfluidic applications before they are transferred to further manipulation steps, like fusion, sorting, exposure to chemicals or others. We use an electrically induced stretching of intact cells. This gives a distinct elongation of different cell lines. As an example system, we present results obtained from two well-established cancerous and non-cancerous cell lines. They differ in the extent of stretching displayed at the same electric forces and in the remanence strain after electric field removal. This deformation reflects differences in the internal cell structure: separate perturbation of cytoskeleton components indicated that the mechanical response in the chosen regime is defined primarily by the microtubule system. The advantage of electric fields for this purpose is that the integration of only two microelectrodes into the microfluidic chips is sufficient. This solution, therefore, is cheap since the respective manufacturing techniques are well established. The electric fields can be controlled finely by appropriate software programs. Overall, these benefits facilitate the transition to an automated and parallelized cell identification module.
Cilia and flagella are beating rod-like organelles that enable the directional movement of microorganisms in fluids and fluid transport along the surface of biological organisms or inside organs. The molecular motor axonemal dynein drives their beating by interacting with microtubules. Constructing synthetic beating systems with axonemal dynein capable of mimicking ciliary beating still represents a major challenge. Here, the bottom-up engineering of a sustained beating synthoneme consisting of a pair of microtubules connected by a series of periodic arrays of approximately eight axonemal dyneins is reported. A model leads to the understanding of the motion through the cooperative, cyclic association–dissociation of the molecular motor from the microtubules. The synthoneme represents a bottom-up self-organized bio-molecular machine at the nanoscale with cilia-like properties.
This paper presents a new framework for particle tracking based on a Gaussian Mixture Model (GMM). It is an extension of the state-of-the-art iterative reconstruction of individual particles by a continuous modeling of the particle trajectories considering the position and velocity as coupled quantities. The proposed approach includes an initialization and a processing step. In the first step, the velocities at the initial points are determined after iterative reconstruction of individual particles of the first four images to be able to generate the tracks between these initial points. From there on, the tracks are extended in the processing step by searching for and including new points obtained from consecutive images based on continuous modeling of the particle trajectories with a Gaussian Mixture Model. The presented tracking procedure allows to extend existing trajectories interactively with low computing effort and to store them in a compact representation using little memory space. To demonstrate the performance and the functionality of this new particle tracking approach, it is successfully applied to a synthetic turbulent pipe flow, to the problem of observing particles corresponding to a Brownian motion (e.g., motion of cells), as well as to problems where the motion is guided by boundary forces, e.g., in the case of particle tracking velocimetry of turbulent Rayleigh–Bénard convection.
Artificial systems capable of self-sustained movement with self-sufficient energy are of high interest with respect to the development of many challenging applications, including medical treatments, but also technical applications. The bottom-up assembly of such systems in the context of synthetic biology is still a challenging task. In this work, we demonstrate the biocompatibility and efficiency of an artificial light-driven energy module and a motility functional unit by integrating light-switchable photosynthetic vesicles with demembranated flagella. The flagellar propulsion is coupled to the beating frequency, and dynamic ATP synthesis in response to illumination allows us to control beating frequency of flagella in a light-dependent manner. In addition, we verified the functionality of light-powered synthetic vesicles in motility assays by encapsulating microtubules assembled with force-generating kinesin-1 motors and the energy module to investigate the dynamics of a contractile filamentous network in cell-like compartments by optical stimulation. Integration of this photosynthetic system with various biological building blocks such as cytoskeletal filaments and molecular motors may contribute to the bottom-up synthesis of artificial cells that are able to undergo motor-driven morphological deformations and exhibit directional motion in a light-controllable fashion.
In nature, interactions between biopolymers and motor proteins give rise to biologically essential emergent behaviors. Besides cytoskeleton mechanics, active nematics arise from such interactions. Here we present a study on 3D active nematics made of microtubules, kinesin motors, and depleting agent. It shows a rich behavior evolving from a nematically ordered space-filling distribution of microtubule bundles toward a flattened and contracted 2D ribbon that undergoes a wrinkling instability and subsequently transitions into a 3D active turbulent state. The wrinkle wavelength is independent of the ATP concentration and our theoretical model describes its relation with the appearance time. We compare the experimental results with a numerical simulation that confirms the key role of kinesin motors in cross-linking and sliding the microtubules. Our results on the active contraction of the network and the independence of wrinkle wavelength on ATP concentration are important steps forward for the understanding of these 3D systems.
Active networks of biopolymers and motor proteins in vitro self-organize and exhibit dynamic structures on length scales much larger than the interacting individual components of which they consist. How the dynamics is related across the range of length scales is still an open question. Here, we experimentally characterize and quantify the dynamic behavior of isolated microtubule bundles that bend due to the activity of motor proteins. At the motor level, we track and describe the motion features of kinesin-1 clusters stepping within the bending bundles. We find that there is a separation of length scales by at least 1 order of magnitude. At a run length of
Shewanella oneidensis MR-1 are facultative aerobic electroactive bacteria with an appealing potential for sustainable energy production and bioremediation. They gather around air sources, forming aerotactic bands and biofilms. Here, we experimentally follow the evolution of the band around an air bubble, and we find good agreement with the numerical solutions of the pertinent transport equations. Video microscopy reveals a transition between motile and non-motile MR-1 upon oxygen depletion, preventing further development of the biofilm. We discover that MR-1 can alternate between longitudinal fast and sideways slow swimming. The resulting bimodal velocity distributions change in response to different oxygen concentrations and gradients, supporting the biological functions of aerotaxis and confinement.
Electroporation is a widely used method for increasing the permeability of the cellular membrane. Impermeant exogenous substances (e.g., DNA, proteins, peptides, dyes) can thus be introduced into the cells. In this study, we have developed an electroporation system for mammalian cells on a microfluidic platform. It is a simple microsystem able to realize permeation processes without using metal electrodes to control the electric field applied to each cell and the pulse duration. In our design, a hydrodynamic focusing of fluids with different conductivities allows for performing electroporation processes in a defined area of the microfluidic channel. Thus, a continuous direct current (DC) is sufficient for supplying the system. No pulse generator or other complex electronic equipment is required. The time for which the cells are exposed to the high electric field is determined by the ratio of the fluid velocities. Electropermeation of yeast cells was observed after applying even comparatively low voltages (
The mechanical behavior of a living cell is highly dynamic and constantly adapts to its local environment. Changes in temperature and chemical stimuli, such as pH, may alter the structure of the cell and its mechanical response. Thus, the mechanical properties may serve as an indicator for the cellular state. We applied dielectrophoretic forces to suspension cells by means of two microelectrodes. The resultant stretching was analyzed on consecutive cultivation days with respect to the influence of medium consumption. Systematic experiments clearly showed that the medium consumption affected the viscoelastic properties of the investigated human leukemia cells HL-60. The shift in pH value and the culture medium depletion were identified as potentially responsible for the differing temporal development of the cell deformation. Both factors were investigated separately and a detailed analysis indicated that the changes observed in the cellular stiffness were primarily attributable to nutrient depletion.
Metal-Induced Energy Transfer (MIET) imaging is an easy-to-implement super-resolution modality that achieves nanometer resolution along the optical axis of a microscope. Although its capability in numerous biological and biophysical studies has been demonstrated, its implementation for live-cell imaging with fluorescent proteins is still lacking. Here, we present its applicability and capabilities for live-cell imaging with fluorescent proteins in diverse cell types (adult human stem cells, human osteo-sarcoma cells, and Dictyostelium discoideum cells), and with various fluorescent proteins (GFP, mScarlet, RFP, YPet). We show that MIET imaging achieves nanometer axial mapping of living cellular and sub-cellular components across multiple timescales, from a few milliseconds to hours, with negligible phototoxic effects. Competing Interest Statement The authors have declared no competing interest.
Kinesin motors can induce a buckling instability in a microtubule with a fixed minus end. Here we show that by modifying the surface with a protein-repellent functionalization and using clusters of kinesin motors, the microtubule can exhibit persistent oscillatory motion resembling the beating of sperm flagella. The observed period is of the order of 1 min. From the experimental images we theoretically determine a distribution of motor forces that explains the observed shapes using a maximum likelihood approach. A good agreement is achieved with a small number of motor clusters acting simultaneously on a microtubule. The tangential forces exerted by a cluster are mostly in the range 0-8 pN toward the microtubule minus end, indicating the action of 1 or 2 kinesin motors. The lateral forces are distributed symmetrically and mainly below 10 pN, while the lateral velocity has a strong peak around zero. Unlike well-known models for flapping filaments, kinesins are found to have a strong "pinning" effect on the beating filaments. Our results suggest new strategies to utilize molecular motors in dynamic roles that depend sensitively on the stress built-up in the system.
Suspensions of microtubules and nonadsorbing particles form thick and long bundles due to depletion forces. Such interactions act at the nanometer scale and define the structural and dynamical properties of the resulting networks. In this study, we analyze the depletion forces exerted by two types of nonadsorbing particles, namely, the polymer, poly(ethylene glycol) (PEG), and the block copolymer, Pluronic. We characterize their effects both in passive and active networks by adding motor proteins to the suspensions. By exploiting its bundling effect via entropic forces, we observed that PEG generates a network with thick structures showing a nematic order and larger mesh size. On the other hand, Pluronic builds up a much denser gel-like network without a recognizable mesh structure. This difference is also reflected in the network activity. PEG networks show moderate contraction in lateral directions while Pluronic networks exhibit faster and isotropic contraction. Interestingly, by mixing the two nonadsorbing polymers in different ratios, we observed that the system showed a behavior that exhibited properties of both agents, leading to a robust and fast responsive structure compared to the single-depletant networks. In conclusion, we show how passive osmotic compression modifies the distribution of biopolymers. Its combination with active motors results in a new active material with potential for nanotechnological applications.
The mechanical behavior of biological cells is mainly determined by the cytoskeleton. Its properties are closely interlinked with many cellular events, including disease-related processes, and, thus, may be exploited as potent biomarkers. We have stretched two types of cells between microelectrodes through the application of dielectrophoretic forces. Small numbers of cells of cancerous origin (MCF-7) and from related noncancerous tissue (MCF-10A) were sufficient to obtain data that allowed us to unambiguously distinguish these cells. The Maxwell tension applied has been estimated to be 56 Pa. A detailed analysis of the cells showed that the differences in the stretching response are due to cell-specific mechanical properties. Through the addition of an actin- and a microtubule-specific toxin to the cells, differences in the microtubular structures of the two cell types have been identified as the major cause for the behavior observed. Our approach shows enormous potential for parallelization and automation. Hence, it should be suitable for achieving throughputs that make it attractive for many biomedical diagnostic purposes.
Cells have the ability to detect electric fields and respond to them with directed migratory movement. Investigations identified genes and proteins that play important roles in defining the migration efficiency. Nevertheless, the sensing and transduction mechanisms underlying directed cell migration are still under discussion. We useDictyostelium discoideumcells as model system for studying eukaryotic cell migration in DC electric fields. We have defined the temporal electric persistence to characterize the memory that cells have in a varying electric field. In addition to imposing a directional bias, we observed that the electric field influences the cellular kinematics by accelerating the movement of cells along their paths. Moreover, the study of vegetative and briefly starved cells provided insight into the electrical sensing of cells. We found evidence that conditioned medium of starved cells was able to trigger the electrical sensing of vegetative cells that would otherwise not orient themselves in the electric field. This observation may be explained by the presence of the conditioned medium factor (CMF), a protein secreted by the cells, when they begin to starve. The results of this study give new insights into understanding the mechanism that triggers the electrical sensing and transduces the external stimulus into directed cell migration. Finally, the observed increased mobility of cells over time in an electric field could offer a novel perspective towards wound healing assays.
Biological cilia pump the surrounding fluid by asymmetric beating that is driven by dynein motors between sliding microtubule doublets. The complexity of biological cilia raises the question about minimal systems that can re-create similar patterns of motion. One such system consists of a pair of microtubules that are clamped at the proximal end. They interact through dynein motors that cover one of the filaments and pull against the other one. Here, we study theoretically the static shapes and the active dynamics of such a system. Using the theory of elastica, we analyse the shapes of two filaments of different lengths with clamped ends. Starting from equal lengths, we observe a transition similar to Euler buckling leading to a planar shape. When further increasing the length ratio, the system assumes a non-planar shape with spontaneously broken chiral symmetry after a secondary bifurcation and then transitions to planar again. The predicted curves agree with experimentally observed shapes of microtubule pairs. The dynamical system can have a stable fixed point, with either bent or straight filaments, or limit cycle oscillations. The latter match many properties of ciliary motility, demonstrating that a two-filament system can serve as a minimal actively beating model.