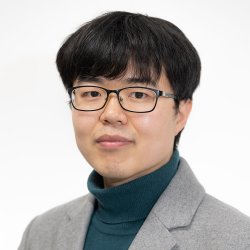
Dr Wooli Bae
About
Biography
Wooli Bae is a Lecturer in Experimental Soft Matter Physics at the University of Surrey.
He received his PhD at KAIST, South Korea in 2014 with his work on single-molecule fluorescence and single-molecule force spectroscopy. After his PhD, he moved to LMU, Germany as a postdoctoral researcher to study DNA nanostructures in the Department of Physics. In 2017, he become a research associate at Imperial College London where he developed RNA reaction circuits for synthetic biology applications. In 2021, he was appointed lecturer in physics at the University of Surrey.
Areas of specialism
My qualifications
Previous roles
ResearchResearch interests
My research area is nucleic acid (NA) nanotechnology where we use DNA and RNA to build nanostructures and reaction circuits. In this highly interdisciplinary field, I use principles of physics to design novel structures and reaction circuits for various applications including information processing, controlling molecular diffusion, plasmonics, molecular sensing and synthetic biology.
My current research topics is using the techniques of NA nanotechnology to build systems that can help us understand fundamental principles of biology. I am constructing a programmable cell mimic using in-situ RNA transcriptional circuits that I developed. Please refer to my Google Scholar profile for a full list of publications.
Research interests
My research area is nucleic acid (NA) nanotechnology where we use DNA and RNA to build nanostructures and reaction circuits. In this highly interdisciplinary field, I use principles of physics to design novel structures and reaction circuits for various applications including information processing, controlling molecular diffusion, plasmonics, molecular sensing and synthetic biology.
My current research topics is using the techniques of NA nanotechnology to build systems that can help us understand fundamental principles of biology. I am constructing a programmable cell mimic using in-situ RNA transcriptional circuits that I developed. Please refer to my Google Scholar profile for a full list of publications.
Sustainable development goals
My research interests are related to the following:
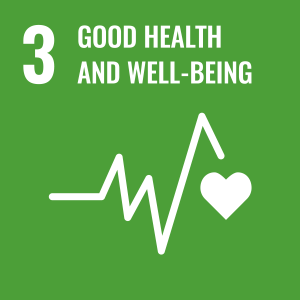
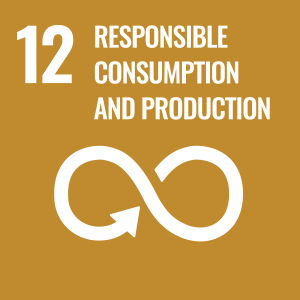
Publications
Oil-in-water emulsions, stabilised with conventional surfactants, are commonly used in eye drops for ocular drug delivery. However, the presence of surfactants can sometimes irritate tissues. Furthermore, conventional emulsions often have poor retention on ocular tissue. Pickering emulsions stabilised with nanoparticles have been gaining attention in recent years for a range of biomedical applications because of their biocompatibility. Here, Pickering emulsions were evaluated for the first time for the confinement of organic components for potential application in ocular drug delivery. For a model system, we used nanodiamond (ND) nanoparticles functionalised with covalently-bonded two-tail (2T) oligoglycine C-10(NGly(4))(2) to make Pickering oil-in-water emulsions, which were stable over three months of storage under neutral pH. We proved the non-toxicity of ND-2T Pickering emulsions, comparable to buffer solution, via an ex vivo bovine corneal permeability and opacity test. The retention of the oil phase in the ND-2T stabilised emulsions on corneal tissue is significantly increased because of the mucoadhesive properties arising from the positively-charged terminal amino groups of 2T. Our formulated emulsions have a surface tension, pH and salt concentration comparable to that of tear fluid. The high retention of the ND-2T-stabilised emulsions on the corneal surface, in combination with their non-toxicity, gives them distinct advantages for ocular drug delivery. The principles of this model system could be applied in the future design of a range of formulations for drug delivery.
Synthetic biology is an interdisciplinary field aiming to design biochemical systems with desired behaviors. To this end, molecular controllers have been developed which, when embedded into a pre-existing ambient biochemical network, control the dynamics of the underlying target molecular species. When integrated into smaller compartments, such as biological cells in vivo, or vesicles in vitro, controllers have to be calibrated to factor in the intrinsic noise. In this context, molecular controllers put forward in the literature have focused on manipulating the mean (first moment), and reducing the variance (second moment), of the target species. However, many critical biochemical processes are realized via higher-order moments, particularly the number and configuration of the modes (maxima) of the probability distributions. To bridge the gap, a controller called stochastic morpher is put forward in this paper, inspired by gene-regulatory networks, which, under suitable time-scale separations, morphs the probability distribution of the target species into a desired predefined form. The morphing can be performed at the lower-resolution, allowing one to achieve desired multi-modality/multi-stability, and at the higher-resolution, allowing one to achieve arbitrary probability distributions. Properties of the controller, such as robust perfect adaptation and convergence, are rigorously established, and demonstrated on various examples. Also proposed is a blueprint for an experimental implementation of stochastic morpher.
DNA nanotechnology offers the possibility to rationally design structures with emergent properties by precisely controlling their geometry and functionality. Here, we demonstrate a DNA-based plasmonic metamolecule that is capable of sensing human thrombin proteins. The chiral reconfigurability of a DNA origami structure carrying two gold nanorods was used to provide optical read-out of thrombin binding through changes in the displayed plasmonic circular dichroism. In our experiments, each arm of the structure was modified with one of two different thrombin-binding aptamers-thrombin-binding aptamer (TBA) and HD22-in such a way that a thrombin molecule could be sandwiched by the aptamers to lock the metamolecule in a state of defined chirality. Our structure exhibited a K-d of 1.4 nM, which was an order of magnitude lower than those of the individual aptamers. The increased sensitivity arose from the avidity gained by the cooperative binding of the two aptamers, which was also reflected by a Hill coefficient of 1.3 +/- 0.3. As we further exploited the strong plasmonic circular dichroism (CD) signals of the metamolecule, our method allowed one-step, high sensitivity optical detection of human thrombin proteins in solution.
Despite the recent development in the design of DNA origami, its folding yet relies on thermal or chemical annealing methods. We here demonstrate mechanical folding of the DNA origami structure via a pathway that has not been accessible to thermal annealing. Using magnetic tweezers, we stretch a single scaffold DNA with mechanical tension to remove its secondary structures, followed by base pairing of the stretched DNA with staple strands. When the force is subsequently quenched, folding of the DNA nanostructure is completed through displacement between the bound staple strands. Each process in the mechanical folding is well defined and free from kinetic traps, enabling us to complete folding within 10 min. We also demonstrate parallel folding of DNA nanostructures through multiplexed manipulation of the scaffold DNAs. Our results suggest a path towards programmability of the folding pathway of DNA nanostructures.
Forces in biological systems are typically investigated at the single-molecule level with atomic force microscopy or optical and magnetic tweezers, but these techniques suffer from limited data throughput and their requirement for a physical connection to the macroscopic world. We introduce a self-assembled nanoscopic force clamp built from DNA that operates autonomously and allows massive parallelization. Single-stranded DNA sections of an origami structure acted as entropic springs and exerted controlled tension in the low piconewton range on a molecular system, whose conformational transitions were monitored by single-molecule Förster resonance energy transfer. We used the conformer switching of a Holliday junction as a benchmark and studied the TATA-binding protein-induced bending of a DNA duplex under tension. The observed suppression of bending above 10 piconewtons provides further evidence of mechanosensitivity in gene regulation.
DNA nanostructures offer the possibility to mimic functional biological membrane components due to their nanometer-precise shape configurability and versatile biochemical functionality. Here we show that the diffusional behavior of DNA nanostructures and their assembly into higher order membrane-bound lattices can be controlled in a stop-and-go manner and that the process can be monitored with super-resolution imaging. The DNA structures are transiently immobilized on glass-supported lipid bilayers by changing the mono- and divalent cation concentrations of the surrounding buffer. Using DNA points accumulation for imaging in nanoscale topography (DNA-PAINT) super-resolution microscopy, we confirm the fixation of DNA origami structures with different shapes. On mica-supported lipid bilayers, in contrast, we observe residual movement. By increasing the concentration of NaCl and depleting MgCl2, a large fraction of DNA structures restarts to diffuse freely on both substrates. After addition of a set of oligonucleotides that enables three Y-shaped monomers to assemble into a three-legged shape (triskelion), the triskelions can be stopped and super-resolved. Exchanging buffer and adding another set of oligonucleotides triggers the triskelions to diffuse and assemble into hexagonal 2D lattices. This stop-and-go imaging technique provides a way to control and observe the diffusional behavior of DNA nanostructures on lipid membranes that could also lead to control of membrane associated cargos.
Biological membranes fulfill many important tasks within living organisms. In addition to separating cellular volumes, membranes confine the space available to membrane-associated proteins to two dimensions (2D), which greatly increases their probability to interact with each other and assemble into multiprotein complexes. We here employed two DNA origami structures functionalized with cholesterol moieties as membrane anchors-a three-layered rectangular block and a Y-shaped DNA structure to mimic membrane-assisted assembly into hierarchical superstructures on supported lipid bilayers and small unilamellar vesicles. As designed, the DNA constructs adhered to the lipid bilayers mediated by the cholesterol anchors and diffused freely in 2D with diffusion coefficients depending on their size and number of cholesterol modifications. Different sets of multimerization oligonucleotides added to bilayer-bound origami block structures induced the growth of either linear polymers or two-dimensional lattices on the membrane. Y-shaped DNA origami structures associated into triskelion homotrimers and further assembled into weakly ordered arrays of-hexagons and pentagons, which resembled the geometry of clathrin-coated pits. Our results demonstrate the potential to realize artificial self-assembling systems that mimic the hierarchical formation of polyhedral lattices on cytoplasmic membranes.
The use of templates is a well-established method for the production of sequence-controlled assemblies, particularly long polymers. Templating is canonically envisioned as akin to a self-assembly process, wherein sequence-specific recognition interactions between a template and a pool of monomers favor the assembly of a particular polymer sequence at equilibrium. However, during the biogenesis of sequence-controlled polymers, template recognition interactions are transient; RNA and proteins detach spontaneously from their templates to perform their biological functions and allow template reuse. Breaking template recognition interactions puts the product sequence distribution far from equilibrium, since specific product formation can no longer rely on an equilibrium dominated by selective copy-template bonds. The rewards of engineering artificial polymer systems capable of spontaneously exhibiting nonequilibrium templating are large, but fields like DNA nanotechnology lack the requisite tools; the specificity and drive of conventional DNA reactions rely on product stability at equilibrium, sequestering any recognition interaction in products. The proposed alternative is handhold-mediated strand displacement (HMSD), a DNA-based reaction mechanism suited to producing out-of-equilibrium products. HMSD decouples the drive and specificity of the reaction by introducing a transient recognition interaction, the handhold. We measure the kinetics of 98 different HMSD systems to prove that handholds can accelerate displacement by 4 orders of magnitude without being sequestered in the final product. We then use HMSD to template the selective assembly of any one product DNA duplex from an ensemble of equally stable alternatives, generating a far-from-equilibrium output. HMSD thus brings DNA nanotechnology closer to the complexity of out-of-equilibrium biological systems.
The quantum yield of a fluorophore is reduced when two or more identical fluorophores are in close proximity to each other. The study of protein folding or particle aggregation is can be done based on this above-mentioned phenomenon-called self-quenching. However, it is challenging to characterize the self-quenching of a fluorophore at high concentrations because of the inner filter effect, which involves depletion of excitation light and re-absorption of emission light. Herein, a novel method to directly evaluate the self-quenching behavior of fluorophores was developed. The evanescent field from an objective-type total internal reflection fluorescence (TIRF) microscope was used to reduce the path length of the excitation and emission light to ~100 nm, thereby supressing the inner filter effect. Fluorescence intensities of sulforhodamine B, fluorescein isothiocyanate (FITC), and calcein solutions with concentrations ranging from 1 μM to 50 mM were directly measured to evaluate the concentration required for 1000-fold degree of self-quenching and to examine the different mechanisms through which the fluorophores undergo self-quenching.
Synthetic RNA systems offer unique advantages such as faster response, increased specificity, and programmability compared to conventional protein-based networks. Here, we demonstrate an in vitro RNA-based toggle switch using RNA aptamers capable of inhibiting the transcriptional activity of T7 or SP6 RNA polymerases. The activities of both polymerases are monitored simultaneously by using Broccoli and malachite green light up aptamer systems. In our toggle switch, a T7 promoter drives the expression of SP6 inhibitory aptamers, and an SP6 promoter expresses T7 inhibitory aptamers. We show that the two distinct states originating from the mutual inhibition of aptamers can be toggled by adding DNA sequences to sequester the RNA inhibitory aptamers. Finally, we assessed our RNA based toggle switch in degrading conditions by introducing controlled degradation of RNAs using a mix of RNases. Our results demonstrate that the RNA-based toggle switch could be used as a control element for nucleic acid networks in synthetic biology applications.
Recent developments in DNA nanotechnology brought rich structural and functional diversity. However, for DNA nanostructures to perform in a biologically relevant context, obstacles such as nuclease activity and low divalent ion concentrations have to be addressed. For drug delivery or gene therapy applications, ultimately the lipid membrane barriers must be targeted and overcome. In this article, we highlight efforts and achievements in enhancing the stability of DNA nanostructures including chemical modifications, covalent crosslinking and coating with protective layers composed of polymers or lipids. We then review interactions between DNA nanostructures and lipid membranes, which are often mediated by ligands for membrane receptors or hydrophobic domains incorporated into the structure. Finally, we present applications of DNA nanostructures on and in lipid membranes, including higher order assembly, controlling membrane curvature, targeting and arranging membrane proteins in living cells and DNA-based synthetic lipid channels. (C) 2019 Elsevier Ltd. All rights reserved.
Membrane fusion is one of the most important cellular processes by which two initially distinct lipid bilayers merge their hydrophobic cores, resulting in one interconnected structure. Proteins, called SNARE (soluble N-ethylmaleimide-sensitive factor-attachment protein receptor), play a central role in the fusion process that is also regulated by several accessory proteins. In order to study the SNARE-mediated membrane fusion, the in vitro protein reconstitution assay involving ensemble FRET (fluorescence resonance energy transfer) has been used over a decade. In this mini-review, we describe several single-molecule-based FRET approaches that have been applied to this field to overcome the shortage of the bulk assay in terms of protein and fusion dynamics.
Multihelical DNA bundles could enhance the functionality of nanomaterials and serve as model architectures to mimic protein filaments on the molecular and cellular level. We report the self-assembly of micrometer-sized helical DNA nanotubes with widely controllable helical diameters ranging from tens of nanometers to a few micrometers. Nanoscale helical shapes of DNA tile tubes (4-, 6-, 8-, 10-, and 12-helix tile tubes) are achieved by introducing discrete amounts of bending and twist through base pair insertions and/or deletions. Microscale helical diameters, which require smaller amounts of twist and bending, are achieved by controlling the intrinsic "supertwist" present in tile tubes with uneven number of helices (11-, 13-, and 15-helix tile tubes). Supertwist fine-tuning also allows us to produce helical nanotubes of defined chirality.
Elaborating efficient strategies and deepening the understanding of light transport at the nanoscale is of great importance for future designs of artificial light-harvesting assemblies and dye-based photonic circuits. In this work, we focus on studying the phenomenon of Forster resonance energy transfer (FRET) among fluorophores of the same kind (homo-FRET) and its implications for energy cascades containing two or three different dye molecules. Utilizing the spatial programmability of DNA origami, we arranged a chain of cyanine 3 (Cy3) dyes flanked at one end with a dye of lower excitation energy, cyanine 5 (Cy5), with or without an additional dye of higher excitation energy, Alexa488, at the other end. We characterized the response of our fluorophore assemblies with bulk and single-molecule spectroscopy and support our measurements by Monte Carlo modeling of energy transfer within the system. We find that, depending on the arrangement of the fluorophores, homo-FRET between the Cy3 dyes can lead to an overall enhanced energy transfer to the acceptor fluorophore. Furthermore, we systematically analyzed the homo-FRET system by addressing the fluorescence lifetime and anisotropy. Finally, we built a homo-FRET-mediated photonic wire capable of transferring energy through the homo-FRET system from the blue donor dye (Alexa488) to the red acceptor fluorophore (Cy5) across a total distance of 16 nm.
Synthetic molecular circuits implementing DNA or RNA strand-displacement reactions can be used to build complex systems such as molecular computers and feedback control systems. Despite recent advances, application of nucleic acid-based circuits in vivo remains challenging due to a lack of efficient methods to produce their essential components, namely, multistranded complexes known as gates, in situ, i.e., in living cells or other autonomous systems. Here, we propose the use of naturally occurring self-cleaving ribozymes to cut a single-stranded RNA transcript into a gate complex of shorter strands, thereby opening new possibilities for the autonomous and continuous production of RNA strands in a stoichiometrically and structurally controlled way.
One of the main objectives of synthetic biology is the development of molecular controllers that can manipulate the dynamics of a given biochemical network that is at most partially known. When integrated into smaller compartments, such as living or synthetic cells, controllers have to be calibrated to factor in the intrinsic noise. In this context, biochemical controllers put forward in the literature have focused on manipulating the mean (first moment) and reducing the variance (second moment) of the target molecular species. However, many critical biochemical processes are realized via higher-order moments, particularly the number and configuration of the probability distribution modes (maxima). To bridge the gap, we put forward the stochastic morpher controller that can, under suitable timescale separations, morph the probability distribution of the target molecular species into a predefined form. The morphing can be performed at a lower-resolution, allowing one to achieve desired multi-modality/multi-stability, and at a higher-resolution, allowing one to achieve arbitrary probability distributions. Properties of the controller, such as robustness and convergence, are rigorously established, and demonstrated on various examples. Also proposed is a blueprint for an experimental implementation of stochastic morpher.
Additional publications
1. Climent-Catala, A., Ouldridge, T. E., Stan, G., Bae, W*. Building an RNA-based Toggle Switch using Inhibitory RNA Aptamers. ACS Synth. Biol. 2022, 11 (2), 562-569.
2. Plesa, T., Stan, G., & Ouldridge, T. E. Bae, W. Quasi-robust control of biochemical reaction networks via stochastic morphing. J. R. Soc. Interface. 2021, 18 (177).
3. Bae, W.*; Yoon, T. Y.; Jeong, C. H. Direct evaluation of self-quenching behavior of fluorophores at high concentrations using an evanescent field. PLOS ONE. 2021, 16(2).
4. Bae, W.; Stan, GB.; Ouldridge, TE. In situ generation of RNA complexes for synthetic molecular strand displacement circuits in autonomous systems. Nano Lett. 2021, 21, 1, 265–271.
5. Cabello-garcia, J., Bae, W., Stan, G. V, & Ouldridge, T. E. Handhold-mediated strand displacement : a nucleic acid-based mechanism for generating far-from-equilibrium assemblies through templated reactions. ACS Nano. 2020, 15, 2, 3272–3283
6. Funck, T.; Liedl, T.; Bae, W*. Dual Aptamer-Functionalized 3D Plasmonic Metamolecule for Thrombin Sensing. Appl. Sci. 2019, 9, 3006.
7. Bae, W.; Kocabey, S.; Liedl, T. DNA Nanostructures in Vitro, in Vivo and on Membranes. Nano Today, 2019, 26, 98–107
8. Kempter, S.; Khmelinskaia, A.; Strauss, M. T.; Schwille, P.; Jungmann, R.; Liedl, T.; Bae, W*. Single Particle Tracking and Super-Resolution Imaging of Membrane-Assisted Stop-and-Go Diffusion and Lattice Assembly of DNA Origami. ACS Nano 2019, 13, 2, 996–1002.
9. Nicoli, F.; Barth, A.; Bae, W.; Neukirchinger, F.; Crevenna, A. H.; Lamb, D. C.; Liedl, T. Directional Photonic Wire Mediated by Homo-Förster Resonance Energy Transfer on a DNA Origami Platform. ACS Nano 2017, 11, 11264–11272.
10. Maier, A. M.; Bae, W.; Schiffels, D.; Emmerig, J. F.; Schiff, M.; Liedl, T. Self-Assembled DNA Tubes Forming Helices of Controlled Diameter and Chirality. ACS Nano 2017, 11, 1301–1306.
11. Nickels, P. C.; Wünsch, B.; Holzmeister, P.; Bae, W.; Kneer, L. M.; Grohmann, D.; Tinnefeld, P.; Liedl, T. Molecular Force Spectroscopy with a DNA Origami-Based Nanoscopic Force Clamp. Science (80-. ). 2016, 354, 305–307.
12. Kocabey, S.; Kempter, S.; List, J.; Xing, Y.; Bae, W.; Schiffels, D.; Shih, W. M.; Simmel, F. C.; Liedl, T. Membrane-Assisted Growth of DNA Origami Nanostructure Arrays. ACS Nano 2015, 9, 3530–3539.
13. Bae, W.; Kim, K.; Min, D.; Ryu, J. K.; Hyeon, C.; Yoon, T. Y. Programmed Folding of DNA Origami Structures through Single-Molecule Force Control. Nat. Commun. 2014, 5, 5654.
14. Bae, W.; Choi, M. G.; Hyeon, C.; Shin, Y. K.; Yoon, T. Y. Real-Time Observation of Multiple-Protein Complex Formation with Single-Molecule FRET. J. Am. Chem. Soc. 2013, 135, 10254–10257.
15. Diao, J.; Ishitsuka, Y.; Bae, W. Single-Molecule FRET Study of SNARE-Mediated Membrane Fusion. Biosci. Rep. 2011, 31, 457–463.