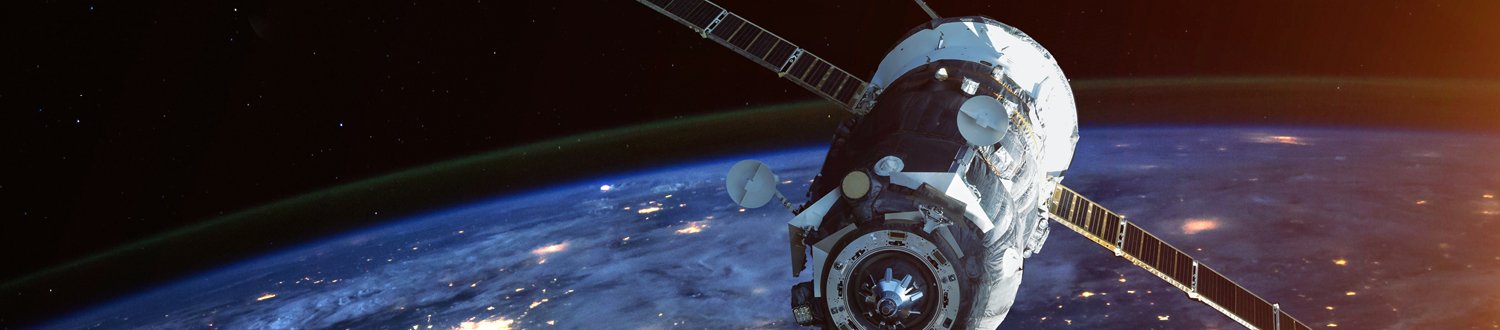
Surrey Space Centre research
Our Centre researches into many varied aspects of satellite design, control and applications. Spacecraft technology requires a broad range of backgrounds from physics and engineering to mathematics and software systems. This broad range is reflected in our research areas which cover many aspects of space and its uses.