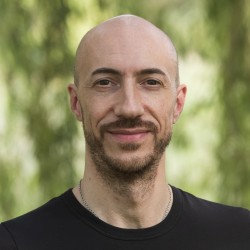
Dr Matteo Barberis FRSB
About
Biography
Matteo started to work on cell cycle control during his master thesis and early PhD in the laboratory of Lilia Alberghina at the University of Milano-Bicocca (Italy), where he was interested to investigate biochemical mechanisms through which cellular activators and inhibitors regulate biochemically the onset into DNA replication in budding yeast. He then moved to the Max Planck Institute for Molecular Genetics in Berlin (Germany), in the laboratory of Edda Klipp, to complete his PhD and translate his experimental research into computational modeling through generation of mathematical models of the eukaryotic cell cycle. He then did a six year Postdoc at both the Max Planck Institute for Molecular Genetics and the Humboldt University in Berlin with Edda Klipp, where he developed as a systems biologist by integrating computational modeling and experimental strategies to uncover novel molecular mechanisms driving a timely cell cycle. In 2013, he then started his independent group as Assistant Professor of Synthetic and Systems Biology at the University of Amsterdam (The Netherlands), where he expanded his investigations to explore how molecular switches drive gene regulation dynamics in cell cycle control and in immune responses, such as those governed by T and mast cells. In December 2018, he joined the University of Surrey as Reader in Systems Biology, with the aim to unravel design principles of cellular organization, and develop multi-scale frameworks to understand how properties of biochemical systems emerge from the integration of multiple regulatory layers. His multi-faceted interests also expands to build cellular maps for network-based drug design, with a particular emphasis on cell physiology and disease.
Altogether, Matteo aims to unravel design principles of cellular organization by integrating computational modeling and molecular biology, in order to predict, test and validate experimentally molecular mechanisms underlying emergent properties of biological systems. He uses yeast and mammalian cells to investigate how dynamics switches timely control the eukaryotic cell division cycle. Furthermore, Matteo is also interested to develop multi-scale frameworks and tools to understand how properties of biological systems emerge from the integration of multiple layers of cellular regulation.
Areas of specialism
News
ResearchResearch interests
Matteo's background integrates Industrial Biotechnology, Biochemistry, Molecular Biology and Computational Modeling. Thanks to this acquired interdisciplinarity, he has embarked in Systems Biology research by integrating experimentation and computation to decipher design principles underlying cellular organization. In his scientific research, Matteo employs modeling methodologies to predict systems properties, which he then tests experimentally.
Matteo has a profound interest in exploring the systems biology approaches to unravel design principles in the life sciences. His research aims, on the one hand, to bring together computation and experimentation to unravel molecular mechanisms, e.g. control of gene regulation, underlying switches responsible for temporal dynamics in cell cycle control and immune responses. On the other hand, he is developing multi-scale frameworks and tools that integrate – by various modeling techniques – layers of cellular regulation, with a particular emphasis on the interface between cell cycle and cell physiology.
Matteo’s researches in Systems Biology involves: (i) integrating experimental and computational approaches to identify design principles underlying cellular organization, (ii) unraveling molecular switches in temporal dynamics, e.g. of cell cycle and of immune responses, (iii) developing multi-scale frameworks and tools that integrate layers of cellular regulation, and (iv) building cellular maps for network-based drug design, with a particular emphasis on cell physiology and disease.
Research interests
Matteo's background integrates Industrial Biotechnology, Biochemistry, Molecular Biology and Computational Modeling. Thanks to this acquired interdisciplinarity, he has embarked in Systems Biology research by integrating experimentation and computation to decipher design principles underlying cellular organization. In his scientific research, Matteo employs modeling methodologies to predict systems properties, which he then tests experimentally.
Matteo has a profound interest in exploring the systems biology approaches to unravel design principles in the life sciences. His research aims, on the one hand, to bring together computation and experimentation to unravel molecular mechanisms, e.g. control of gene regulation, underlying switches responsible for temporal dynamics in cell cycle control and immune responses. On the other hand, he is developing multi-scale frameworks and tools that integrate – by various modeling techniques – layers of cellular regulation, with a particular emphasis on the interface between cell cycle and cell physiology.
Matteo’s researches in Systems Biology involves: (i) integrating experimental and computational approaches to identify design principles underlying cellular organization, (ii) unraveling molecular switches in temporal dynamics, e.g. of cell cycle and of immune responses, (iii) developing multi-scale frameworks and tools that integrate layers of cellular regulation, and (iv) building cellular maps for network-based drug design, with a particular emphasis on cell physiology and disease.
Publications
Anxiety disorders disproportionally affect females and are frequently comorbid with eating disorders. With the emerging field of nutritional psychiatry, focus has been put on the impact of diet quality in anxiety pathophysiology and gut microbiome underlying mechanisms. While the relationship between diet and anxiety is bidirectional, improving dietary habits could better facilitate the actions of pharmacological and psychological therapies, or prevent their use. A better understanding of how gut bacteria mediate and moderate such relationship could further contribute to develop personalized programs and inform probiotics and prebiotics manufacturing. To date, studies that look simultaneously at diet, the gut microbiome, and anxiety are missing as only pairwise relationships among them have been investigated. Therefore, this study aims at summarizing and integrating the existing knowledge on the dietary effects on anxiety with focus on gut microbiome. Findings on the effects of diet on anxiety are critically summarized and reinterpreted in relation to findings on (i) the effects of diet on the gut microbiome composition, and (ii) the associations between the abundance of certain gut bacteria and anxiety. This novel interpretation suggests a theoretical model where the relationship between diet and anxiety is mediated and/or modulated by the gut microbiome through multiple mechanisms. In parallel, this study critically evaluates methodologies employed in the nutritional field to investigate the effects of diet on anxiety highlighting a lack of systematic operationalization and assessment strategies. Therefore, it ultimately proposes a novel evidence-based approach that can enhance studies validity, reliability, systematicity, and translation to clinical and community settings.
PI4KA-related disorder is a highly clinically variable condition characterized by neurological (limb spasticity, developmental delay, intellectual disability, seizures, ataxia, nystagmus) and gastrointestinal (inflammatory bowel disease and multiple intestinal atresia) manifestations. Although features consistent with immunodeficiency (autoimmunity/autoinflammation and recurrent infections) have been reported in a subset of patients, the burden of B-cell deficiency and hypogammaglobulinemia has not been extensively investigated. We sought to describe the clinical presentation and manifestations of patients with PI4KA-related disorder and to investigate the metabolic consequences of biallelic PI4KA variants in B cells. Clinical data from patients with PI4KA variants were obtained. Multi-omics analyses combining transcriptome, proteome, lipidome and metabolome analyses in conjunction with functional assays were performed in EBV-transformed B cells. Clinical and laboratory data of 13 patients were collected. Recurrent infections (7/13), autoimmune/autoinflammatory manifestations (5/13), B-cell deficiency (8/13) and hypogammaglobulinemia (8/13) were frequently observed. Patients' B cells frequently showed increased transitional and decreased switched memory B-cell subsets. Pathway analyses based on differentially expressed transcripts and proteins confirmed the central role of PI4KA in B cell differentiation with altered B-cell receptor (BCR) complex and signalling. By altering lipids production and tricarboxylic acid cycle regulation, and causing increased endoplasmic reticulum stress, biallelic PI4KA mutations disrupt B cell metabolism inducing mitochondrial dysfunction. As a result, B cells show hyperactive PI3K/mTOR pathway, increased autophagy and deranged cytoskeleton organization. By altering lipid metabolism and TCA cycle, impairing mitochondrial activity, hyperactivating mTOR pathway and increasing autophagy, PI4KA-related disorder causes a syndromic inborn error of immunity presenting with B-cell deficiency and hypogammaglobulinemia.
The development of T follicular helper (Tfh) cells is an ongoing process resulting in the formation of various Tfh subsets. Despite advancements, the precise impact of T cell receptor (TCR) stimulation on this process remains incompletely understood. This study explores how TCR-CD3 signaling strength influences naive CD4 T cell differentiation into Tfh-like cells and the concurrent expression of interleukin-21 (IL-21), interleukin-4 (IL-4), and interferon-gamma (IFN-γ). Strong TCR-CD3 stimulation induces proliferation and increased IL-21 expression in Tfh-like cells, which exhibit a characteristic phenotype expressing CXCR5 and PD1. The coexpression of IL-4 and IFN-γ in IL-21-producing Tfh-like cells is controlled by the strength TCR-CD3 stimulation; low stimulation favors IL-4, while strong stimulation enhances IFN-γ secretion. Exogenous addition of the effector cytokines IL-21 and IL-4 further modulate cytokine coexpression. These findings highlight the intricate regulatory mechanisms governing cytokine production and plasticity in Tfh-like cells, providing insights into B cell response modulation. , antigen availability may regulate Tfh cell plasticity, impacting subsequent B cell differentiation, emphasizing the need for further exploration through animal models or antigen-specific Tfh cell analyses in human lymph node biopsies.
Coordination of cell cycle with metabolism exists in all cell types that grow by division. It serves to build a new cell, (i) fueling building blocks for the synthesis of proteins, nucleic acids, and membranes, and (ii) producing energy through glycolysis. Cyclin-dependent kinases (Cdks) play an essential role in this coordination, thereby in the regulation of cell division. Cdks are functional homologs across eukaryotes and are the engines that drive cell cycle events and the clocks that time them. Their function is counteracted by stoichiometric inhibitors; specifically, inhibitors of cyclin-cyclin dependent kinase (cyclin/Cdk) complexes allow for their activity at specific times. Here, we provide a new perspective about the yet unknown cell cycle mechanisms impacting on metabolism. We first investigated the effect of the mitotic cyclin/Cdk1 complex Cyclin B/Cdk1-functional homolog in mammalian cells of the budding yeast Clb2/Cdk1-on yeast metabolic enzymes of, or related to, the glycolysis pathway. Six glycolytic enzymes (Glk1, Hxk2, Pgi1, Fba1, Tdh1, and Pgk1) were subjected to in vitro Cdk-mediated phosphorylation assays. Glucose-6-phosphate dehydrogenase (Zwf1), the first enzyme in the pentose phosphate pathway that is important for NADPH production, and 6-phospho-fructo-2-kinase (Pfk27), which catalyzes fructose-2,6-bisphosphate synthesis, a key regulator of glycolysis, were also included in the study. We found that, among these metabolic enzymes, Fba1 and Pgk1 may be phosphorylated by Cdk1, in addition to the known Cdk1-mediated phosphorylation of Gph1. We then investigated the possible effect of Sic1, stoichiometric inhibitor of mitotic cyclin/Cdk1 complexes in budding yeast, on the activities of three most relevant glycolytic enzymes: Hxk2, Glk1, and Tdh1. We found that Sic1 may have a negative effect on Hxk2. Altogether, we reveal possible new routes, to be further explored, through which cell cycle may regulate cellular metabolism. Because of the functional homology of cyclin/Cdk complexes and their stoichiometric inhibitors across evolution, our findings may be relevant for the regulation of cell division in eukaryotes.
Coordination of cell cycle and metabolism exists in all cells. The building of a new cell is a process that requires metabolic commitment to the provision of both Gibbs energy and building blocks for proteins, nucleic acids, and membranes. On the other hand, the cell cycle machinery will assess and regulate its metabolic environment before it makes decisions on when to enter the next cell cycle phase. Furthermore, more and more evidence demonstrate that the metabolism can be regulated by cell cycle progression, as different biosynthesis pathways are preferentially active in different cell cycle phases. Here, we review the available literature providing a critical overview on how cell cycle and metabolism may be coupled with one other, bidirectionally, in the budding yeast Saccharomyces cerevisiae .
Conceptual scheme of the strategy to integrate Genome–Scale Metabolic Models and Flux Balance Analysis for studying, designing, and testing novel probiotics. [Display omitted] •Metabolic diversity of microbial communities allows for widespread applications.•Genome–Scale Metabolic Models are useful tools for studying microbial communities.•Flux Balance Analysis arises as a promising tool for probiotics design and testing.•Metabolic modeling is used for testing probiotics in inflammatory disorders.•Probiotics can complement treatments to increase effectiveness and life quality. The importance of bacterial communities has been acknowledged over the past decades. Their applications span from the study of their role in ecosystems, the use of biofilms in nature and industry, the use of bacterial cultures for biotechnological purposes (such as the production of compounds and photovoltaic energy, and bioremediation), to their investigation in human health, particularly the gut microbiome, for the modulation of the metabolism and the immune system. Here we propose a perspective highlighting the importance of engineering microbial communities to leverage Genome-Scale Metabolic Models (GSMMs) for testing probiotics with therapeutic potential. Specifically, we envision that multispecies genome-scale community metabolic models can be employed to decipher metabolic routes for probiotic and prebiotic production to treat inflammatory diseases.
The interplay between the immune system and the metabolic state of a cell is intricate. In all phases of an immune response, the corresponding metabolic changes shall occur to support its modulation, in addition to the signalling through the cytokine environment and immune receptor stimulation. While autoimmune disorders may develop because of a metabolic imbalance that modulates switching between T‐cell phenotypes, the effects that the interaction between T and B cells have on one another's cellular metabolism are yet to be understood in disease context. Here, we propose a perspective which highlights the potential of targeting metabolism to modulate T‐ and B‐cell subtypes populations as well as T–B and B–T cell interactions to successfully treat autoimmune disorders. Specifically, we envision how metabolic changes can tip the balance of immune cells interactions, through definite mechanisms in both health and disease, to explain phenotype switches of B and T cells. Within this scenario, we highlight targeting metabolism that link inflammation, immunometabolism, epigenetics and ageing, is critical to understand inflammatory disorders. The combination of treatments targeting immune cells that cause (T/B) cell phenotype imbalances, and the metabolic pathways involved, may increase the effectiveness of treatment of autoimmune disorders, and/or ameliorate their symptoms to improve patients’ quality of life. Metabolic imbalance underlies development of autoimmune disorders. Metabolic changes modulate switching between immune cell phenotypes. Targeting metabolism regulates T–B and B–T cell interactions. Ageing at the interface between autoimmune disorders and metabolic syndromes. Conceptual scheme of T–B and B–T cell interactions at the metabolic level. The mutual modulation of metabolic pathways between T and B cells favours an increased metabolic activity, generating feedback of activation. T cells can affect B‐cell metabolism and B cells can affect T‐cell metabolism, through the signalling pathways triggered both by receptor stimulation and cytokine release. Through mutual regulation, T and B cells can meet the metabolic demand required in each stage of their development and differentiate to a specific phenotype.
Biological functions require a coherent cross talk among multiple layers of regulation within the cell. Computational efforts that aim to understand how these layers are integrated across spatial, temporal, and functional scales represent a challenge in Systems Biology. We have developed a computational, multi-scale framework that couples cell cycle and metabolism networks in the budding yeast cell. Here we describe the methodology at the basis of this framework, which integrates on off-the-shelf logical (Boolean) models of a minimal yeast cell cycle with a constraint-based model of metabolism (i.e., the Yeast 7 metabolic network reconstruction). Models are implemented in Python code using the BooleanNet and COBRApy packages, respectively, and are connected through the Boolean logic. The methodology allows for incorporation of interaction data, and validation through -omics data. Furthermore, evolutionary strategies may be incorporated to explore regulatory structures underlying coherent cross talks among regulatory layers.
The budding yeast genome comprises roughly 6000 genes generating a number of about 10000 mRNA copies, which gives a general estimation of 1-2 mRNA copies generated per gene. What does this observation implicate for cellular processes and their regulation? Whether the number of mRNA molecules produced is important for setting the amount of proteins implicated in a particular function is at present unknown. In this context, we studied cell cycle control as one of the highly fine tuned processes that guarantee the precise timing of events essential for cell growth. Here, we developed a stochastic model that addresses the effect of varying the mRNA amount of Sic1, inhibitor of the Cdk1-Clb5 kinase activity, and the resulting noise on Sic1/Clb5 balance at the G1/S transition. We considered a range of SIC1 transcripts number according to our experimental data derived from the MS2 mRNA tagging system. Computational simulation revealed that an increased amount of SIC1 mRNAs lead to an amplified dispersion of Sic1 protein levels, suggesting mRNA control being critical to set timing of Sic1 downregulation and, therefore, S phase onset. Moreover, Sic1/Clb5 balance is strongly influenced by Clb5 production in both daughter and mother cells in order to maintain the characteristic time of S phase entry overall the population. Furthermore, CLB5 mRNA molecules calculated to reproduce temporal dynamics of Sic1 and Clb5 for daughter and mother cells agree with recent data obtained from more complex networks. Thus, the results presented here provide novel insights into the influence that the mRNA amount and, indirectly, the transcription process exploit on cell cycle progression. © 2011 The Royal Society of Chemistry.
Similarly to metazoans, the budding yeast Saccharomyces cereviasiae replicates its genome with a defined timing. In this organism, well-defined, site-specific origins, are efficient and fire in almost every round of DNA replication. However, this strategy is neither conserved in the fission yeast Saccharomyces pombe, nor in Xenopus or Drosophila embryos, nor in higher eukaryotes, in which DNA replication initiates asynchronously throughout S phase at random sites. Temporal and spatial controls can contribute to the timing of replication such as Cdk activity, origin localization, epigenetic status or gene expression. However, a debate is going on to answer the question how individual origins are selected to fire in budding yeast. Two opposing theories were proposed: the "replicon paradigm" or "temporal program" vs. the "stochastic firing". Recent data support the temporal regulation of origin activation, clustering origins into temporal blocks of early and late replication. Contrarily, strong evidences suggest that stochastic processes acting on origins can generate the observed kinetics of replication without requiring a temporal order. In mammalian cells, a spatiotemporal model that accounts for a partially deterministic and partially stochastic order of DNA replication has been proposed. Is this strategy the solution to reconcile the conundrum of having both organized replication timing and stochastic origin firing also for budding yeast? In this review we discuss this possibility in the light of our recent study on the origin activation, suggesting that there might be a stochastic component in the temporal activation of the replication origins, especially under perturbed conditions.
Cell cycle control is highly regulated to guarantee the precise timing of events essential for cell growth, i.e., DNA replication onset and cell division. Failure of this control plays a role in cancer and molecules called cyclin-dependent kinase (Cdk) inhibitors (Ckis) exploit a critical function in cell cycle timing. Here we present a multiscale modeling where experimental and computational studies have been employed to investigate structure, function and temporal dynamics of the Cki Sic1 that regulates cell cycle progression in Saccharomyces cerevisiae. Structural analyses reveal molecular details of the interaction between Sic1 and Cdk/cyclin complexes, and biochemical investigation reveals Sic1 function in analogy to its human counterpart p27Kip1, whose deregulation leads to failure in timing of kinase activation and, therefore, to cancer. Following these findings, a bottom-up systems biology approach has been developed to characterize modular networks addressing Sic1 regulatory function. Through complementary experimentation and modeling, we suggest a mechanism that underlies Sic1 function in controlling temporal waves of cyclins to ensure correct timing of the phase-specific Cdk activities. © 2012 Springer Science+Business Media, LLC.
The identification of large regulatory and signalling networks involved in the control of crucial cellular processes calls for proper modelling approaches. Indeed, models can help elucidate properties of these networks, understand their behaviour and provide (testable) predictions by performing in silico experiments. In this context, qualitative, logical frameworks have emerged as relevant approaches, as demonstrated by a growing number of published models, along with new methodologies and software tools. This productive activity now requires a concerted effort to ensure model reusability and interoperability between tools. Following an outline of the logical modelling framework, we present the most important achievements of the Consortium for Logical Models and Tools, along with future objectives. Our aim is to advertise this open community, which welcomes contributions from all researchers interested in logical modelling or in related mathematical and computational developments. © The Author 2014.
Systems Biology brings the potential to discover fundamental principles of Life that cannot be discovered by considering individual molecules. This chapter discusses a number of early, more recent, and upcoming discoveries of such network principles. These range from the balancing of fluxes through metabolic networks, the potential of those networks for truly individualized medicine, the time dependent control of fluxes and concentrations in metabolism and signal transduction, the ways in which organisms appear to regulate metabolic processes vis-à -vis limitations therein, tradeoffs in robustness and fragility, and a relation between robustness and time dependences in the cell cycle. The robustness considerations will lead to the issue whether and how evolution has been able to put in place design principles of control engineering such as infinite robustness and perfect adaptation in the hierarchical biochemical networks of cell biology © 2014 Elsevier Inc. All rights reserved.
We have previously demonstrated that the cyclin-dependent kinase inhibitor (Cki) Sic1 of Saccharomyces cerevisiae is phosphorylated in vitro by the CK2 kinase on Ser201 residue. Moreover, we have collected evidence showing that Sic1 is functionally and structurally related to mammalian Cki p27Kip1 and binds to the mammalian Cdk2/cyclin A complex with a similar mode of inhibition. In this paper, we use SPR analysis to investigate the binding of Sic1 to the catatytic and regulatory subunits of CK2. Evidence is presented showing that phosphorylation of Sic1 at the CK2 consensus site QES201EDEED increases the binding of a Sic1-derived peptide to the Cdk2/cyclin A complex, a functional homologue of the yeast Cdk1/Clb5,6. Moreover, Sic1 fully phosphorylated in vitro on Ser201 by CK2 is shown to be a stronger inhibitor of the Cdk/cyclin complexes than the unphosphorylated protein. Taken together, these data disclose the possibility that CK2 plays a role in the regulation of Sic1 activity.
CD4+ T cells provide adaptive immunity against pathogens and abnormal cells, and they are also associated with various immune-related diseases. CD4+ T cells’ metabolism is dysregulated in these pathologies and represents an opportunity for drug discovery and development. Genome-scale metabolic modeling offers an opportunity to accelerate drug discovery by providing high-quality information about possible target space in the context of a modeled disease. Here, we develop genome-scale models of naïve, Th1, Th2, and Th17 CD4+ T-cell subtypes to map metabolic perturbations in rheumatoid arthritis, multiple sclerosis, and primary biliary cholangitis. We subjected these models to in silico simulations for drug response analysis of existing FDA-approved drugs and compounds. Integration of disease-specific differentially expressed genes with altered reactions in response to metabolic perturbations identified 68 drug targets for the three autoimmune diseases. In vitro experimental validation, together with literature-based evidence, showed that modulation of fifty percent of identified drug targets suppressed CD4+ T cells, further increasing their potential impact as therapeutic interventions. Our approach can be generalized in the context of other diseases, and the metabolic models can be further used to dissect CD4+ T-cell metabolism.
Sirtuins are a family of highly conserved NAD+-dependent proteins and this dependency links Sirtuins directly to metabolism. Sirtuins’ activity has been shown to extend the lifespan of several organisms and mainly through the post-translational modification of their many target proteins, with deacetylation being the most common modification. The seven mammalian Sirtuins, SIRT1 through SIRT7, have been implicated in regulating physiological responses to metabolism and stress by acting as nutrient sensors, linking environmental and nutrient signals to mammalian metabolic homeostasis. Furthermore, mammalian Sirtuins have been implicated in playing major roles in mammalian pathophysiological conditions such as inflammation, obesity and cancer. Mammalian Sirtuins are expressed heterogeneously among different organs and tissues, and the same holds true for their substrates. Thus, the function of mammalian Sirtuins together with their substrates is expected to vary among tissues. Any therapy depending on Sirtuins could therefore have different local as well as systemic effects. Here, an introduction to processes relevant for the actions of Sirtuins, such as metabolism and cell cycle, will be followed by reasoning on the system-level function of Sirtuins and their substrates in different mammalian tissues. Their involvement in the healthy metabolism and metabolic disorders will be reviewed and critically discussed.
Abstract Computational models of biological systems can exploit a broad range of rapidly developing approaches, including novel experimental approaches, bioinformatics data analysis, emerging modelling paradigms, data standards and algorithms. A discussion about the most recent advances among experts from various domains is crucial to foster data-driven computational modelling and its growing use in assessing and predicting the behaviour of biological systems. Intending to encourage the development of tools, approaches and predictive models, and to deepen our understanding of biological systems, the Community of Special Interest (COSI) was launched in Computational Modelling of Biological Systems (SysMod) in 2016. SysMod’s main activity is an annual meeting at the Intelligent Systems for Molecular Biology (ISMB) conference, which brings together computer scientists, biologists, mathematicians, engineers, computational and systems biologists. In the five years since its inception, SysMod has evolved into a dynamic and expanding community, as the increasing number of contributions and participants illustrate. SysMod maintains several online resources to facilitate interaction among the community members, including an online forum, a calendar of relevant meetings and a YouTube channel with talks and lectures of interest for the modelling community. For more than half a decade, the growing interest in computational systems modelling and multi-scale data integration has inspired and supported the SysMod community. Its members get progressively more involved and actively contribute to the annual COSI meeting and several related community workshops and meetings, focusing on specific topics, including particular techniques for computational modelling or standardisation efforts.
Biological circuits are responsible for transitions between cellular states in a timely fashion. For example, stem cells switch from an undifferentiated (unstable) state to a differentiated (stable) state. Conversely, cell cycle and circadian clocks are completed through transitions among successive (stable) states, i.e. waves, with (unstable) states switching them at definite timing. These transitions irreversibly determine the biological response or fate of a cell, to commit to reversible switches or to generate periodic oscillations of its state. Here, we review synthetic circuits that, in silico and in vivo, allow a cell to ‘make a decision’, i.e. to select which state to reach, among multiple ones available, through definite network designs. Specifically, we propose and discuss the designs, and their constituents motifs, which we consider to be more prone to reprogram cell behaviour, and whose parameters can be fine-tuned through systems biology and tested experimentally through Synthetic Biology. For these designs, exploration of the parameter space and of the influence of (external) cellular signals – which modulate circuit parameters – allows for the prediction of the circuit's response and its consequent impact on cell fate.
After two decades of exciting progress, innovative research in synthetic biology has transformed the work of systems biology, metabolic engineering, and biotechnology. The field's research includes many notable achievements, such as bacteria performing complex computation, yeast cells manufacturing complex drugs, and human cells designed to correct genetic diseases. Programming living cells with synthetic genes to perform useful new functions has even become a routine achievement for summer school students.
Precise timing of cell division is achieved by coupling waves of cyclin-dependent kinase (Cdk) activity with a transcriptional oscillator throughout cell cycle progression. Although details of transcription of cyclin genes are known, it is unclear which is the transcriptional cascade that modulates their expression in a timely fashion. Here, we demonstrate that a Clb/Cdk1-mediated regulation of the Fkh2 transcription factor synchronizes the temporal mitotic CLB expression in budding yeast. A simplified kinetic model of the cyclin/Cdk network predicts a linear cascade where a Clb/Cdk1-mediated regulation of an activator molecule drives CLB3 and CLB2 expression. Experimental validation highlights Fkh2 as modulator of CLB3 transcript levels, besides its role in regulating CLB2 expression. A Boolean model based on the minimal number of interactions needed to capture the information flow of the Clb/Cdk1 network supports the role of an activator molecule in the sequential activation, and oscillatory behavior, of mitotic Clb cyclins. This work illustrates how transcription and phosphorylation networks can be coupled by a Clb/Cdk1-mediated regulation that synchronizes them. © 2017, The Author(s).
A number of techniques have been developed in order to address issues such as genome, trascriptome and proteome analysis. However, a time and cost effective technique for interactome analysis is still lacking. Lots of methods for the predicion of protein - protein interacions have been developed: some of them are based on high quality alignment of sequences, others are based on the tridimensional features of proteins, but they all bear strong limitations that make impossible their large scale application. Recently, an SVM-based machine learning approach has been used to address this topic. Although the method was able to correctly classify 80% of the test samples, it was not applied to the prediction of yet unknown interactions. In this work, we address this topic and show that an optimized, SVM-based machine learning approach trained with combinations of shuffled sequences as examples of lack of interaction is unable to make large scale predictions of interaction. © Springer-Verlag Berlin Heidelberg 2003.
We present a systems biology view on pseudoenzymes that acknowledges that genes are not selfish: the genome is. With network function as the selectable unit, there has been an evolutionary bonus for recombination of functions of and within proteins. Many proteins house a functionality by which they 'read' the cell's state, and one by which they 'write' and thereby change that state. Should the writer domain lose its cognate function, a 'pseudoenzyme' or 'pseudosignaler' arises. GlnK involved in Escherichia coli ammonia assimilation may well be a pseudosignaler, associating 'reading' the nitrogen state of the cell to 'writing' the ammonium uptake activity. We identify functional pseudosignalers in the cyclin-dependent kinase complexes regulating cell-cycle progression. For the mitogen-activated protein kinase pathway, we illustrate how a 'dead' pseudosignaler could produce potentially selectable functionalities. Four billion years ago, bioenergetics may have shuffled 'electron-writers', producing various networks that all served the same function of anaerobic ATP synthesis and carbon assimilation from hydrogen and carbon dioxide, but at different ATP/acetate ratios. This would have enabled organisms to deal with variable challenges of energy need and substrate supply. The same principle might enable 'gear-shifting' in real time, by dynamically generating different pseudo-redox enzymes, reshuffling their coenzymes, and rerouting network fluxes. Non-stationary pH gradients in thermal vents together with similar such shuffling mechanisms may have produced a first selectable proton-motivated pyrophosphate synthase and subsequent ATP synthase. A combination of functionalities into enzymes, signalers, and the pseudoversions thereof may offer fitness in terms of plasticity, both in real time and in evolution.
We here apply a control analysis and various types of stability analysis to an in silico model of innate immunity that addresses the management of inflammation by a therapeutic peptide. Motivation is the observation, both in silico and in experiments, that this therapy is not robust. Our modeling results demonstrate how (1) the biological phenomena of acute and chronic modes of inflammation may reflect an inherently complex bistability with an irrevertible flip between the two modes, (2) the chronic mode of the model has stable, sometimes unique, steady states, while its acute-mode steady states are stable but not unique, (3) as witnessed by TNF levels, acute inflammation is controlled by multiple processes, whereas its chronic-mode inflammation is only controlled by TNF synthesis and washout, (4) only when the antigen load is close to the acute mode's flipping point, many processes impact very strongly on cells and cytokines, (5) there is no antigen exposure level below which reduction of the antigen load alone initiates a flip back to the acute mode, and (6) adding healthy fibroblasts makes the transition from acute to chronic inflammation revertible, although (7) there is a window of antigen load where such a therapy cannot be effective. This suggests that triple therapies may be essential to overcome chronic inflammation. These may comprise (1) anti-immunoglobulin light chain peptides, (2) a temporarily reduced antigen load, and (3a) fibroblast repopulation or (3b) stem cell strategies.
DNA replication is restricted to a specific time window of the cell cycle, called S phase. Successful progression through S phase requires replication to be properly regulated to ensure that the entire genome is duplicated exactly once, without errors, in a timely fashion. As a result, DNA replication has evolved into a tightly regulated process involving the coordinated action of numerous factors that function in all phases of the cell cycle. Biochemical mechanisms driving the eukaryotic cell division cycle have been the subject of a number of mathematical models. However, cell cycle networks reported in literature so far have not addressed the steps of DNA replication events. In particular, the assembly of the replication machinery is crucial for the timing of S phase. This event, called "initiation", which occurs in late M / early G1 of the cell cycle, starts with the assembly of the pre-replicative complex (pre-RC) at the origins of replication on the DNA. Its activation depends on the availability of different kinase complexes, cyclin-dependent kinases (CDKs) and Dbf-dependent kinase (DDK), which phosphorylate specific components of the pre-RC to convert it into the pre-initiation complex (pre-IC). We have developed an ODE-based model of the network responsible for this process in budding yeast by using mass-action kinetics. We considered all steps from the assembly of the first components at the DNA replication origin up to the active replisome that recruits the polymerases and verified the computational dynamics with the available literature data. Our results highlighted the link between activation of CDK and DDK and the step-by-step formation of both pre-RC and pre-IC, suggesting S-CDK (Cdk1-Clb5,6) to be the main regulator of the process.
The understanding of complex biological processes whose function requires the interaction of a large number of components is strongly improved by the construction of mathematical models able to capture the underlying regulatory wirings and to predict the dynamics of the process in a variety of conditions. Iterative rounds of simulations and experimental analysis generate models of increasing accuracy, what is called the systems biology approach. The cell cycle is one of the complex biological processes that benefit from this approach, and in particular budding yeast is an established model organism for these studies. The recent publication about the modeling of the G1/S transition of the budding yeast cell cycle under a systems biology analysis has highlighted in particular the implications of the cell size determination that impinge the events driving DNA replication. During the life cycle of eukaryotic cells, DNA replication is restricted to a specific time window, called the S phase, and several control mechanisms ensure that each DNA sequence is replicated once, and only once, in the period from one cell division to the next. Here we extend the analysis of the G1/S transition model by including additional aspects concerning the DNA replication process, in order to give a reasonable explanation to the experimental dynamics, as well as of specific cell cycle mutants. Moreover, we show the mathematical description of the critical cell mass (Ps) that cells have to reach to start DNA replication, which value is modulated depending on the different activation of the replication origins. The sensitivity analysis of the influence that the kinetic parameters of the G1/S transition model have on the setting of the Ps value is also reported.
Motivation Multi-scale modeling of biological systems requires integration of various information about genes and proteins that are connected together in networks. Spatial, temporal and functional information is available; however, it is still a challenge to retrieve and explore this knowledge in an integrated, quick and user-friendly manner. Results We present GEMMER (GEnome-wide tool for Multi-scale Modeling data Extraction and Representation), a web-based data-integration tool that facilitates high quality visualization of physical, regulatory and genetic interactions between proteins/genes in Saccharomyces cerevisiae. GEMMER creates network visualizations that integrate information on function, temporal expression, localization and abundance from various existing databases. GEMMER supports modeling efforts by effortlessly gathering this information and providing convenient export options for images and their underlying data. Availability and implementation GEMMER is freely available at http://gemmer.barberislab.com. Source code, written in Python, JavaScript library D3js, PHP and JSON, is freely available at https://github.com/barberislab/GEMMER.
The understanding of complex biological processes whose function requires the interaction of a large number of components is strongly improved by the construction of mathematical models able to capture the underlying regulatory wirings and to predict the dynamics of the process in a variety of conditions. Iterative rounds of simulations and experimental analysis generate models of increasing accuracy, what is called the systems biology approach. The cell cycle is one of the complex biological processes that benefit from this approach, and in particular budding yeast is an established model organism for these studies. The recent publication about the modeling of the G(1)/S transition of the budding yeast cell cycle under a systems biology analysis has highlighted in particular the implications of the cell size determination that impinge the events driving DNA replication. During the life cycle of eukaryotic cells, DNA replication is restricted to a specific time window, called the S phase, and several control mechanisms ensure that each DNA sequence is replicated once, and only once, in the period from one cell division to the next. Here we extend the analysis of the G(1)/S transition model by including additional aspects concerning the DNA replication process, in order to give a reasonable explanation to the experimental dynamics, as well as of specific cell cycle mutants. Moreover, we show the mathematical description of the critical cell mass (P-S) that cells have to reach to start DNA replication, which value is modulated depending on the different activation of the replication origins. The sensitivity analysis of the influence that the kinetic parameters of the G(1)/S transition model have on the setting of the P-S value is also reported.
Cellular systems biology aims to uncover design principles that describe the properties of biological networks through interaction of their components in space and time. The cell cycle is a complex system regulated by molecules that are integrated into functional modules to ensure genome integrity and faithful cell division. In budding yeast, cyclin-dependent kinases (Cdk1/Clb) drive cell cycle progression, being activated and inactivated in a precise temporal sequence. In this module, which we refer to as the 'Clb module', different Cdk1/Clb complexes are regulated to generate waves of Clb activity, a functional property of cell cycle control. The inhibitor Sic1 plays a critical role in the Clb module by binding to and blocking Cdk1/Clb activity, ultimately setting the timing of DNA replication and mitosis. Fifteen years of research subsequent to the identification of Sic1 have lead to the development of an integrative approach that addresses its role in regulating the Clb module. Sic1 is an intrinsically disordered protein and achieves its inhibitory function by cooperative binding, where different structural regions stretch on the Cdk1/Clb surface. Moreover, Sic1 promotes S phase entry, facilitating Cdk1/Clb5 nuclear transport, and therefore revealing a double function of inhibitor/activator that rationalizes a mechanism to prevent precocious DNA replication. Interestingly, the investigation of Clb temporal dynamics by mathematical modelling and experimental validation provides evidence that Sic1 acts as a timer to coordinate oscillations of Clb cyclin waves. Here we review these findings, focusing on the design principle underlying the Clb module, which highlights the role of Sic1 in regulating phase-specific Cdk1/Clb activities.
With genome sequencing of thousands of organisms, a scaffold has become available for data integration: molecular information can now be organized by attaching it to the genes and their gene-expression products. It is however, the genome that is selfish not the gene, making it necessary to organize the information into maps that enable functional interpretation of the fitness of the genome. Using flux balance analysis one can calculate the theoretical capabilities of the living organism. Here we examine whether according to this genome organized information, organisms such as the ones present when life on Earth began, are able to assimilate the Gibbs energy and carbon that life needs for its reproduction and maintenance, from a relatively poor Gibbs-energy environment. We shall address how Clostridium ljungdahlii may use at least two special features and one special pathway to this end: gear-shifting, electron bifurcation and the Wood-Ljungdahl pathway. Additionally, we examined whether the C. ljungdahlii map can also help solve the problem of waste management. We find that there is a definite effect of the choices of redox equivalents in the Wood-Ljungdahl pathway and the hydrogenase on the yield of interesting products like hydroxybutyrate. We provide a drawing of a subset of the metabolic network that may be utilized to project flux distributions onto by the community in future works. Furthermore, we make all the code leading to the results discussed here publicly available for the benefit of future work.
Mathematical models are key to systems biology where they typically describe the topology and dynamics of biological networks, listing biochemical entities and their relationships with one another. Some (hyper)thermophilic Archaea contain an enzyme, called non-phosphorylating glyceraldehyde-3-phosphate dehydrogenase (GAPN), which catalyzes the direct oxidation of glyceraldehyde-3-phosphate to 3-phosphoglycerate omitting adenosine 5′-triphosphate (ATP) formation by substrate-level-phosphorylation via phosphoglycerate kinase. In this study we formulate three hypotheses that could explain functionally why GAPN exists in these Archaea, and then construct and use mathematical models to test these three hypotheses. We used kinetic parameters of enzymes of Sulfolobus solfataricus (S. solfataricus) which is a thermo-acidophilic archaeon that grows optimally between 60 and 90 °C and between pH 2 and 4. For comparison, we used a model of Saccharomyces cerevisiae (S. cerevisiae), an organism that can live at moderate temperatures. We find that both the first hypothesis, i.e., that the glyceraldehyde-3-phosphate dehydrogenase (GAPDH) plus phosphoglycerate kinase (PGK) route (the alternative to GAPN) is thermodynamically too much uphill and the third hypothesis, i.e., that GAPDH plus PGK are required to carry the flux in the gluconeogenic direction, are correct. The second hypothesis, i.e., that the GAPDH plus PGK route delivers less than the 1 ATP per pyruvate that is delivered by the GAPN route, is only correct when GAPDH reaction has a high rate and 1,3-bis-phosphoglycerate (BPG) spontaneously degrades to 3PG at a high rate.
Background: DNA replication begins at specific locations called replication origins, where helicase and polymerase act in concert to unwind and process the single DNA filaments. The sites of active DNA synthesis are called replication forks. The density of initiation events is low when replication forks travel fast, and is high when forks travel slowly. Despite the potential involvement of epigenetic factors, transcriptional regulation and nucleotide availability, the causes of differences in replication times during DNA synthesis have not been established satisfactorily, yet. Methodology/Principal Findings: Here, we aimed at quantifying to which extent sequence properties contribute to the DNA replication time in budding yeast. We interpreted the movement of the replication machinery along the DNA template as a directed random walk, decomposing influences on DNA replication time into sequence-specific and sequence independent components. We found that for a large part of the genome the elongation time can be well described by a global average replication rate, thus by a single parameter. However, we also showed that there are regions within the genomic landscape of budding yeast with highly specific replication rates, which cannot be explained by global properties of the replication machinery. Conclusion/Significance: Computational models of DNA replication in budding yeast that can predict replication dynamics have rarely been developed yet. We show here that even beyond the level of initiation there are effects governing the replication time that can not be explained by the movement of the polymerase along the DNA template alone. This allows us to characterize genomic regions with significantly altered elongation characteristics, independent of initiation times or sequence composition
The Forkhead (Fkh) box family of transcription factors is evolutionary conserved from yeast to higher eukaryotes and its members are involved in many physiological processes including metabolism, DNA repair, cell cycle, stress resistance, apoptosis, and aging. In budding yeast, four Fkh transcription factors were identified, namely Fkh1, Fkh2, Fhl1, and Hcm1, which are implicated in chromatin silencing, cell cycle regulation, and stress response. These factors impinge transcriptional regulation during cell cycle progression, and histone deacetylases (HDACs) play an essential role in this process, e.g., the nuclear localization of Hcm1 depends on Sir2 activity, whereas Sin3/Rpd3 silence cell cycle specific gene transcription in G2/M phase. However, a direct involvement of Sir2 in Fkh1/Fkh2-dependent regulation of target genes is at present unknown. Here, we show that Fkh1 and Fkh2 associate with Sir2 in G1 and M phase, and that Fkh1/Fkh2-mediated activation of reporter genes is antagonized by Sir2. We further report that Sir2 overexpression strongly affects cell growth in an Fkh1/Fkh2-dependent manner. In addition, Sir2 regulates the expression of the mitotic cyclin Clb2 through Fkh1/Fkh2-mediated binding to the CLB2 promoter in G1 and M phase. We finally demonstrate that Sir2 is also enriched at the CLB2 promoter under stress conditions, and that the nuclear localization of Sir2 is dependent on Fkh1 and Fkh2. Taken together, our results show a functional interplay between Fkh1/Fkh2 and Sir2 suggesting a novel mechanism of cell cycle repression. Thus, in budding yeast, not only the regulation of G2/M gene expression but also the protective response against stress could be directly coordinated by Fkh1 and Fkh2. © 2013 Linke, Klipp, Lehrach, Barberis and Krobitsch.
Triggering an appropriate protective response against invading agents is crucial to the effectiveness of human innate and adaptive immunity. Pathogen recognition and elimination requires integration of a myriad of signals from many different immune cells. For example, T cell functioning is not qualitatively, but quantitatively determined by cellular and humoral signals. Tipping the balance of signals, such that one of these is favored or gains advantage on another one, may impact the plasticity of T cells. This may lead to switching their phenotypes and, ultimately, modulating the balance between proliferating and memory T cells to sustain an appropriate immune response. We hypothesize that, similar to other intracellular processes such as the cell cycle, the process of T cell differentiation is the result of: (i) pleiotropy (pattern) and (ii) magnitude (dosage/concentration) of input signals, as well as (iii) their timing and duration. That is, a flexible, yet robust immune response upon recognition of the pathogen may result from the integration of signals at the right dosage and timing. To investigate and understand how system's properties such as T cell plasticity and T cell-mediated robust response arise from the interplay between these signals, the use of experimental toolboxes that modulate immune proteins may be explored. Currently available methodologies to engineer T cells and a recently devised strategy to measure protein dosage may be employed to precisely determine, for example, the expression of transcription factors responsible for T cell differentiation into various subtypes. Thus, the immune response may be systematically investigated quantitatively. Here, we provide a perspective of how pattern, dosage and timing of specific signals, called interleukins, may influence T cell activation and differentiation during the course of the immune response. We further propose that interleukins alone cannot explain the phenotype variability observed in T cells. Specifically, we provide evidence that the dosage of intercellular components of both the immune system and the cell cycle regulating cell proliferation may contribute to T cell activation, differentiation, as well as T cell memory formation and maintenance. Altogether, we envision that a qualitative (pattern) and quantitative (dosage) crosstalk between the extracellular milieu and intracellular proteins leads to T cell plasticity and robustness. The understanding of this complex interplay is crucial to predict and prevent scenarios where tipping the balance of signals may be compromised, such as in autoimmunity.
Budding yeast cell cycle oscillates between states of low and high cyclin-dependent kinase activity, driven by association of Cdk1 with B-type (Clb) cyclins. Various Cdk1-Clb complexes are activated and inactivated in a fixed, temporally regulated sequence, inducing the behaviour known as "waves of cyclins". The transition from low to high Clb activity is triggered by degradation of Sic1, the inhibitor of Cdk1-Clb complexes, at the entry to S phase. The G 1 phase is characterized by low Clb activity and high Sic1 levels. High Clb activity and Sic1 proteolysis are found from the beginning of the S phase until the end of mitosis. The mechanism regulating the appearance on schedule of Cdk1-Clb complexes is currently unknown. Here, we analyse oscillations of Clbs, focusing on the role of their inhibitor Sic1. We compare mathematical networks differing in interactions that Sic1 may establish with Cdk1-Clb complexes. Our analysis suggests that the wave-like cyclins pattern derives from the binding of Sic1 to all Clb pairs rather than from Clb degradation. These predictions are experimentally validated, showing that Sic1 indeed interacts and coexists in time with Clbs. Intriguingly, a sic1δ strain looses cell cycle-regulated periodicity of Clbs, which is observed in the wild type, whether a SIC1-0P strain delays the formation of Clb waves. Our results highlight an additional role for Sic1 in regulating Cdk1-Clb complexes, coordinating their appearance.
The eukaryotic cell cycle is robustly designed, with interacting molecules organized within a definite topology that ensures temporal precision of its phase transitions. Its underlying dynamics are regulated by molecular switches, for which remarkable insights have been provided by genetic and molecular biology efforts. In a number of cases, this information has been made predictive, through computational models. These models have allowed for the identification of novel molecular mechanisms, later validated experimentally. Logical modeling represents one of the youngest approaches to address cell cycle regulation. We summarize the advances that this type of modeling has achieved to reproduce and predict cell cycle dynamics. Furthermore, we present the challenge that this type of modeling is now ready to tackle: its integration with intracellular networks, and its formalisms, to understand crosstalks underlying systems level properties, ultimate aim of multi-scale models. Specifically, we discuss and illustrate how such an integration may be realized, by integrating a minimal logical model of the cell cycle with a metabolic network. © FEMS 2017. All rights reserved.
Many a disease associates with inflammation. Upon binding of antigen-antibody complexes to immunoglobulin-like receptors, mast cells release tumor necrosis factor-α and proteases, causing fibroblasts to release endogenous antigens that may be cross reactive with exogenous antigens. We made a predictive dynamic map of the corresponding extracellular network. In silico, this map cleared bacterial infections, via acute inflammation, but could also cause chronic inflammation. In the calculations, limited inflammation flipped to strong inflammation when cross-reacting antigen exceeded an "On threshold." Subsequent reduction of the antigen load to below this "On threshold" did not remove the strong inflammation phenotype unless the antigen load dropped below a much lower and subtler "Off" threshold. In between both thresholds, the network appeared caught either in a "low" or a "high" inflammatory state. This was not simply a matter of bi-stability, however, the transition to the "high" state was temporarily revertible but ultimately irreversible: removing antigen after high exposure reduced the inflammatory phenotype back to "low" levels but if then the antigen dosage was increased only a little, the high inflammation state was already re-attained. This property may explain why the high inflammation state is indeed "chronic," whereas only the naive low-inflammation state is "acute." The model demonstrates that therapies of chronic inflammation such as with anti-IgLC should require fibroblast implantation (or corresponding stem cell activation) for permanence in order to redress the irreversible transition. © 2018 Abudukelimu, Barberis, Redegeld, Sahin and Westerhoff.
The Community of Special Interest (COSI) in Computational Modelling of Biological Systems (SysMod) brings together interdisciplinary scientists interested in combining data-driven computational modelling, multi-scale mechanistic frameworks, large-scale -omics data and bioinformatics. SysMod's main activity is an annual meeting at the Intelligent Systems for Molecular Biology (ISMB) conference, a meeting for computer scientists, biologists, mathematicians, engineers and computational and systems biologists. The 2021 SysMod meeting was conducted virtually due to the ongoing COVID-19 pandemic (coronavirus disease 2019). During the 2-day meeting, the development of computational tools, approaches and predictive models was discussed, along with their application to biological systems, emphasizing disease mechanisms. This report summarizes the meeting.
Forkhead Box (Fox) proteins share the Forkhead domain, a wingedhelix DNA binding module, which is conserved among eukaryotes from yeast to humans. These sequence-specific DNA binding proteins have been primarily characterized as transcription factors regulating diverse cellular processes from cell cycle control to developmental fate, deregulation of which contributes to developmental defects, cancer, and aging. We recently identified Saccharomyces cerevisiae Forkhead 1 (Fkh1) and Forkhead 2 (Fkh2) as required for the clustering of a subset of replication origins in G1 phase and for the early initiation of these origins in the ensuing S phase, suggesting a mechanistic role linking the spatial organization of the origins and their activity. Here, we show that Fkh1 and Fkh2 share a unique structural feature of human FoxP proteins that enables FoxP2 and FoxP3 to form domain-swapped dimers capable of bridging two DNA molecules in vitro. Accordingly, Fkh1 self-associates in vitro and in vivo in a manner dependent on the conserved domain-swapping region, strongly suggestive of homodimer formation. Fkh1- and Fkh2-domain-swap-minus (dsm) mutations are functional as transcription factors yet are defective in replication origin timing control. Fkh1-dsm binds replication origins in vivo but fails to cluster them, supporting the conclusion that Fkh1 and Fkh2 dimers perform a structural role in the spatial organization of chromosomal elements with functional importance.
Some biological networks exhibit oscillations in their components to convert stimuli to time-dependent responses. The eukaryoticcell cycle is such a case, being governed by waves of cyclin-dependent kinase (cyclin/Cdk) activities that rise and fall with specifictiming and guarantee its timely occurrence. Disruption of cyclin/Cdk oscillations could result in dysfunction through reduced celldivision. Therefore, it is of interest to capture properties of network designs that exhibit robust oscillations. Here we show that aminimal yeast cell cycle network is able to oscillate autonomously, and that cyclin/Cdk-mediated positive feedback loops (PFLs) andClb3-centered regulations sustain cyclin/Cdk oscillations, in known and hypothetical network designs. We propose that Clb3-mediated coordination of cyclin/Cdk waves reconciles checkpoint and oscillatory cell cycle models. Considering the evolutionaryconservation of the cyclin/Cdk network across eukaryotes, we hypothesize that functional (“healthy”) phenotypes require thecapacity to oscillate autonomously whereas dysfunctional (potentially“diseased”) phenotypes may lack this capacity.
Activation (in the following referred to as firing) of replication origins is a continuous and irreversible process regulated by availability of DNA replication molecules and cyclin-dependent kinase activities, which are often altered in human cancers. The temporal, progressive origin firing throughout S phase appears as a characteristic replication profile, and computational models have been developed to describe this process. Although evidence from yeast to human indicates that a range of replication fork rates is observed experimentally in order to complete a timely S phase, those models incorporate velocities that are uniform across the genome. Taking advantage of the availability of replication profiles, chromosomal position and replication timing, here we investigated how fork rate may affect origin firing in budding yeast. Our analysis suggested that patterns of origin firing can be observed from a modulation of the fork rate that strongly correlates with origin density. Replication profiles of chromosomes with a low origin density were fitted with a variable fork rate, whereas for the ones with a high origin density a constant fork rate was appropriate. This indeed supports the previously reported correlation between inter-origin distance and fork rate changes. Intriguingly, the calculated correlation between fork rate and timing of origin firing allowed the estimation of firing efficiencies for the replication origins. This approach correctly retrieved origin efficiencies previously determined for chromosome VI and provided testable prediction for other chromosomal origins. Our results gain deeper insights into the temporal coordination of genome duplication, indicating that control of the replication fork rate is required for the timely origin firing during S phase.
Background: The cell cycle is a complex process that allows eukaryotic cells to replicate chromosomal DNA and partition it into two daughter cells. A relevant regulatory step is in the G0/G1phase, a point called the restriction (R) point where intracellular and extracellular signals are monitored and integrated. Results: Subcellular localization of cell cycle proteins is increasingly recognized as a major factor that regulates cell cycle transitions. Nevertheless, current mathematical models of the G1/S networks of mammalian cells do not consider this aspect. Hence, there is a need for a computational model that incorporates this regulatory aspect that has a relevant role in cancer, since altered localization of key cell cycle players, notably of inhibitors of cyclin-dependent kinases, has been reported to occur in neoplastic cells and to be linked to cancer aggressiveness. Conclusion: The network of the model components involved in the G1to S transition process was identified through a literature and web-based data mining and the corresponding wiring diagram of the G1to S transition drawn with Cell Designer notation. The model has been implemented in Mathematica using Ordinary Differential Equations. Time-courses of level and of sub-cellular localization of key cell cycle players in mouse fibroblasts re-entering the cell cycle after serum starvation/re-feeding have been used to constrain network design and parameter determination. The model allows to recapitulate events from growth factor stimulation to the onset of S phase. The R point estimated by simulation is consistent with the R point experimentally determined. The major element of novelty of our model of the G1to S transition is the explicit modeling of cytoplasmic/nuclear shuttling of cyclins, cyclin-dependent kinases, their inhibitor and complexes. Sensitivity analysis of the network performance newly reveals that the biological effect brought about by Cki overexpression is strictly dependent on whether the Cki is promoting nuclear translocation of cyclin/Cdk containing complexes. © 2009 Alfieri et al; licensee BioMed Central Ltd.
A pharmacology that hits single disease-causing molecules with a single drug passively distributing to the target tissue, was almost ready. Such a pharmacology is not (going to be) effective however: a great many diseases are systems biology diseases; complex networks of some hundred thousand types of molecule, determine the functions that constitute human health, through nonlinear interactions. Malfunctions are caused by a variety of molecular failures at the same time; rarely the same variety in different individuals; in complex constellations of OR and AND logics. Few molecules cause disease single-handedly and few drugs will cure the disease all by themselves when dosed for a limited amount of time. We here discuss the implications that this discovery of the network nature of disease should have for pharmacology. We suggest ways in which pharmacokinetics, pharmacodynamics, but also systems biology and genomics may have to change so as better to deal with systems-biology diseases.
The immune system is highly complex, and its malfunctioning can result in many complex disorders. Understanding its inner workings is crucial to designing optimal immunotherapies, developing new vaccines, or understanding autoimmune diseases, just to name a few. Immune-related diseases present unique challenges due to our limited understanding of the complex molecular and cellular interactions involved, as well as the scarcity of available therapeutic options. Recent years have witnessed the progressive development of high-throughput experimental technologies to probe the immune system. This large amount of data has facilitated the emergence of statistical and machine-learning models focused on unravelling the intricate complexities of the immune system. With this vision in mind, a workshop titled "Computational modelling of immunological mechanisms: From statistical approaches to interpretable machine learning" was organized on Sunday, September 18th, 2022 at the 21st European Conference on Computational Biology (ECCB) in Sitges, Spain. The workshop, led by María Rodríguez Martínez, Anna Niarakis, and Matteo Barberis, explored recent statistical models, high-throughput data analyses, and machine learning models to understand immunological mechanisms. More than 60 participants attended the workshop, comprising students, early-career and senior researchers, as well as professionals from diverse domains including Immunology, Systems Biology, Computational Biology, Computer Science, and Bioinformatics. To conclude the workshop, a round table was organized to foster discussions on the existing challenges and chart a roadmap for the development of the next generation of computational models dedicated to investigating the cellular and molecular functions that underlie the immune system.
How the network around ROS protects against oxidative stress and Parkinson’s disease (PD), and how processes at the minutes timescale cause disease and aging after decades, remains enigmatic. Challenging whether the ROS network is as complex as it seems, we built a fairly comprehensive version thereof which we disentangled into a hierarchy of only five simpler subnetworks each delivering one type of robustness. The comprehensive dynamic model described in vitro data sets from two independent laboratories. Notwithstanding its five-fold robustness, it exhibited a relatively sudden breakdown, after some 80 years of virtually steady performance: it predicted aging. PD-related conditions such as lack of DJ-1 protein or increased α-synuclein accelerated the collapse, while antioxidants or caffeine retarded it. Introducing a new concept (aging-time-control coefficient), we found that as many as 25 out of 57 molecular processes controlled aging. We identified new targets for “life-extending interventions”: mitochondrial synthesis, KEAP1 degradation, and p62 metabolism.
In budding yeast, synchronization of waves of mitotic cyclins that activate the Cdk1 kinase occur through Forkhead transcription factors. These molecules act as controllers of their sequential order and may account for the separation in time of incompatible processes. Here, a Forkhead-mediated design principle underlying the quantitative model of Cdk control is proposed for budding yeast. This design rationalizes timing of cell division, through progressive and coordinated cyclin/Cdk-mediated phosphorylation of Forkhead, and autonomous cyclin/Cdk oscillations. A “ clock unit ” incorporating this design that regulates timing of cell division is proposed for both yeast and mammals, and has a DRIVER operating the incompatible processes that is instructed by multiple CLOCKS. TIMERS determine whether the clocks are active, whereas CONTROLLERS determine how quickly the clocks shall function depending on external MODULATORS. This “ clock unit ” may coordinate temporal waves of cyclin/Cdk concentration/activity in the eukaryotic cell cycle making the driver operate the incompatible processes, at separate times.
Twenty years ago, the term ‘Systems Biology’ has been coined, after which Institutes of Systems Biology were established in Tokyo, Seattle and Amsterdam by the pioneers Hiroaki Kitano, Leroy Hood, and Hans Westerhoff, respectively. For their achievements, in 2014 they did receive the recognition of ‘Fellows of The International Society for Systems Biology’. Through their work, and that of their collaborators, the field has progressively matured as an important lever to make biology predictive, testable and quantitative. Systems Biology in combination with Bioinformatics and high-throughput experiments turns data analysis into a forecasting tool. Systems Biology in combination with imaging allows us to understand processes in cells in two or three or even four dimensions.
Cellular growth is ensured by alternation of DNA duplication and cell division cycles. This alternation is coordinated by the interplay between enzymatic activities, called kinases, and transcription factors, to keep the cell cycle timing. Here we investigate whether transcription factors may serve as hubs connecting multi-scale cellular networks. A variant of chromatin immunoprecipitation, called ChIP-exo, was performed to identify targets of Forkhead (Fkh) transcription factors across the budding yeast genome. Data analyses indicate that the Fkh-mediated transcriptional program may activate metabolic pathways and synchronize kinase activities to guarantee alternation of DNA duplication and cell division, thereby a timely cell’s cycling.
In Saccharomyces cerevisiae, Sic1, an inhibitor of Cdk (cyclin-dependent kinase), blocks the activity of S-Cdk1 (Cdk1/Clb5,6) kinase that is required for DNA replication. Deletion of Sic1 causes premature DNA replication from fewer origins, extension of the S phase and inefficient separation of sister chromatids during anaphase. Despite the well-documented relevance of Sic1 inhibition of S-Cdk1 for cell cycle control and genome instability, the molecular mechanism by which Sic1 inhibits S-Cdk1 activity remains obscure. In this paper, we show that Sicl is functionally and structurally related to the mammalian Cki (Cdk inhibitor) p27Kip1 of the Kip/Cip family. A molecular model of the inhibitory domain of Sic1 bound to the Cdk2-cyclin A complex suggested that the yeast inhibitor might productively interface with the mammalian Cdk2-cyclin A complex. Consistent with this, Sic1 is able to bind to, and strongly inhibit the kinase activity of, the Cdk2-cyclin A complex. In addition, comparison of the different inhibitory patterns obtained using histone H1 or GST (glutathione S-transferase)-pRb (retinoblastoma protein) fusion protein as substrate (the latter of which recognizes both the docking site and the catalytic site of Cdk2-cyclin A) offers interesting suggestions for the inhibitory mechanism of Sic1. Finally, overexpression of the KIP1 gene in vivo in Saccharomyces cerevisiae, like overexpression of the related SIC1 gene, rescues the cell cycle-related phenotype of a sic1Δ strain. Taken together, these findings strongly indicate that budding yeast Sic1 and mammalian p27Kip1 are functional homologues with a structurally conserved inhibitory domain.
The understanding of the multi-scale nature of molecular networks represents a major challenge. For example, regulation of a timely cell cycle must be coordinated with growth, during which changes in metabolism occur, and integrate information from the extracellular environment, e.g. signal transduction. Forkhead transcription factors are evolutionarily conserved among eukaryotes, and coordinate a timely cell cycle progression in budding yeast. Specifically, Fkh1 and Fkh2 are expressed during a lengthy window of the cell cycle, thus are potentially able to function as hubs in the multi-scale cellular environment that interlocks various biochemical networks. Here we report on a novel ChIP-exo dataset for Fkh1 and Fkh2 in both logarithmic and stationary phases, which is analyzed by novel and existing software tools. Our analysis confirms known Forkhead targets from available ChIP-chip studies and highlights novel ones involved in the cell cycle, metabolism and signal transduction. Target genes are analyzed with respect to their function, temporal expression during the cell cycle, correlation with Fkh1 and Fkh2 as well as signaling and metabolic pathways they occur in. Furthermore, differences in targets between Fkh1 and Fkh2 are presented. Our work highlights Forkhead transcription factors as hubs that integrate multi-scale networks to achieve proper timing of cell division in budding yeast.
The eukaryotic cell cycle is the repeated sequence of events that enable the division of a cell into two daughter cells. It is divided into four phases: G1, S, G2, and M. Passage through the cell cycle is strictly regulated by a molecular interaction network, which involves the periodic synthesis and destruction of cyclins that bind and activate cyclin-dependent kinases that are present in nonlimiting amounts. Cyclin-dependent kinase inhibitors contribute to cell cycle control. Budding yeast is an established model organism for cell cycle studies, and several mathematical models have been proposed for its cell cycle. An area of major relevance in cell cycle control is the G1 to S transition. In any given growth condition, it is characterized by the requirement of a specific, critical cell size, PS, to enter S phase. The molecular basis of this control is still under discussion. The authors report a mathematical model of the G1 to S network that newly takes into account nucleo/cytoplasmic localization, the role of the cyclin-dependent kinase Sic1 in facilitating nuclear import of its cognate Cdk1-Clb5, Whi5 control, and carbon source regulation of Sic1 and Sic1-containing complexes. The model was implemented by a set of ordinary differential equations that describe the temporal change of the concentration of the involved proteins and protein complexes. The model was tested by simulation in several genetic and nutritional setups and was found to be neatly consistent with experimental data. To estimate PS, the authors developed a hybrid model including a probabilistic component for firing of DNA replication origins. Sensitivity analysis of PS provides a novel relevant conclusion: PS is an emergent property of the G1 to S network that strongly depends on growth rate.
Network complexity is required to lend cellular processes flexibility to respond timely to a variety of dynamic signals, while simultaneously warranting robustness to protect cellular integrity against perturbations. The cell cycle serves as a paradigm for such processes; it maintains its frequency and temporal structure (although these may differ among cell types) under the former, but accelerates under the latter. Cell cycle molecules act together in time and in different cellular compartments to execute cell type-specific programs. Strikingly, the timing at which molecular switches occur is controlled by abundance and stoichiometry of multiple proteins within complexes. However, traditional methods that investigate one effector at a time are insufficient to understand how modulation of protein complex dynamics at cell cycle transitions shapes responsiveness, yet preserving robustness. To overcome this shortcoming, we propose a multidisciplinary approach to gain a systems-level understanding of quantitative cell cycle dynamics in mammalian cells from a new perspective. By suggesting advanced experimental technologies and dedicated modeling approaches, we present innovative strategies (i) to measure absolute protein concentration in vivo, and (ii) to determine how protein dosage, e.g., altered protein abundance, and spatial (de)regulation may affect timing and robustness of phase transitions. We describe a method that we name “Maximum Allowable mammalian Trade–Off–Weight” (MAmTOW), which may be realized to determine the upper limit of gene copy numbers in mammalian cells. These aspects, not covered by current systems biology approaches, are essential requirements to generate precise computational models and identify (sub)network-centered nodes underlying a plethora of pathological conditions.
Sic1, cyclin-dependent kinase inhibitor of budding yeast, is synthesized in anaphase and largely degraded at the S-phase onset to regulate timing of DNA synthesis. Sic1 interacts with phase-specific B-type cyclin (Clb)-kinase (Cdk1) complexes, central regulators in cell cycle control. Its appearance is timed to mediate reduction in kinase activities at appropriate stages. Clbs are unstable proteins with extremely short half-lives. Interactions of Sic1 with Clbs have been detected both in vitro and in vivo by high-throughput genome-wide screenings. Furthermore, we have recently shown that Sic1 regulates waves of Clbs, acting as a timer in their appearance, thus controlling Cdk1-Clbs activation. The molecular mechanism is not yet fully understood but is hypothesized to occur via stoichiometric binding of Sic1 to Cdk1-Clb complexes. Using Förster resonance energy transfer (FRET) via fluorescence lifetime imaging microscopy (FLIM), we showed association of Sic1 to Clb cyclins in living yeast cells. This finding is consistent with the notion that inhibition of kinase activity can occur over the whole cell cycle progression despite variable Sic1 levels. Specifically, Sic1/Clb3 interaction was observed for the first time, and Sic1/Clb2 and Sic1/Clb5 pairs were confirmed, but no Sic1/Clb4 interaction was found, which suggests that, despite high functional homology between Clbs, only some of them can target Sic1 function in vivo.
DNA replication in eukaryotes is considered to proceed according to a precise program in which each chromosomal region is duplicated in a defined temporal order. However, recent studies reveal an intrinsic temporal disorder in the replication of yeast chromosome VI. Here we provide a model of the chromosomal duplication to study the temporal sequence of origin activation in budding yeast. The model comprises four parameters that influence the DNA replication system: the lengths of the chromosomes, the explicit chromosomal positions for all replication origins as well as their distinct initiation times and the replication fork migration rate. The designed model is able to reproduce the available experimental data in form of replication profiles. The dynamics of DNA replication was monitored during simulations of wild type and randomly perturbed replication conditions. Severe loss of origin function showed only little influence on the replication dynamics, so systematic deletions of origins (or loss of efficiency) were simulated to provide predictions to be tested experimentally. The simulations provide new insights into the complex system of DNA replication, showing that the system is robust to perturbation, and giving hints about the influence of a possible disordered firing.
CD4+ T cells provide cell-mediated immunity in response to various antigens. During an immune response, naïve CD4+ T cells differentiate into specialized effector T helper (Th1, Th2, and Th17) cells and induced regulatory (iTreg) cells based on a cytokine milieu. In recent studies, complex phenotypes resembling more than one classical T cell lineage have been experimentally observed. Herein, we sought to characterize the capacity of T cell differentiation in response to the complex extracellular environment. We constructed a comprehensive mechanistic (logical) computational model of the signal transduction that regulates T cell differentiation. The model's dynamics were characterized and analyzed under 511 different environmental conditions. Under these conditions, the model predicted the classical as well as the novel complex (mixed) T cell phenotypes that can co-express transcription factors (TFs) related to multiple differentiated T cell lineages. Analyses of the model suggest that the lineage decision is regulated by both compositions and dosage of signals that constitute the extracellular environment. In this regard, we first characterized the specific patterns of extracellular environments that result in novel T cell phenotypes. Next, we predicted the inputs that can regulate the transition between the canonical and complex T cell phenotypes in a dose-dependent manner. Finally, we predicted the optimal levels of inputs that can simultaneously maximize the activity of multiple lineage-specifying TFs and that can drive a phenotype toward one of the co-expressed TFs. In conclusion, our study provides new insights into the plasticity of CD4+ T cell differentiation, and also acts as a tool to design testable hypotheses for the generation of complex T cell phenotypes by various input combinations and dosages. © 2018 Puniya, Todd, Mohammed, Brown, Barberis and Helikar.
Computer-aided design (CAD) for synthetic biology promises to accelerate the rational and robust engineering of biological systems. It requires both detailed and quantitative mathematical and experimental models of the processes to (re)design biology, and software and tools for genetic engineering and DNA assembly. Ultimately, the increased precision in the design phase will have a dramatic impact on the production of designer cells and organisms with bespoke functions and increased modularity. CAD strategies require quantitative models of cells that can capture multiscale processes and link genotypes to phenotypes. Here, we present a perspective on how whole-cell, multiscale models could transform design-build-test-learn cycles in synthetic biology. We show how these models could significantly aid in the design and learn phases while reducing experimental testing by presenting case studies spanning from genome minimization to cell-free systems. We also discuss several challenges for the realization of our vision. The possibility to describe and build whole-cells in silico offers an opportunity to develop increasingly automatized, precise and accessible CAD tools and strategies.