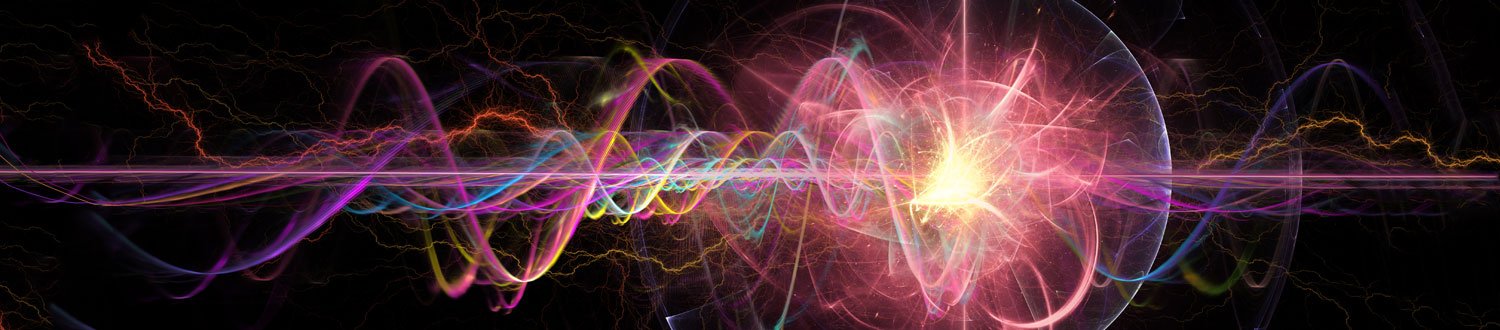
Quantum thermodynamics and open quantum systems
Quantum thermodynamics is a relatively new area of fundamental research and a promising avenue of study. The extension of classical thermodynamic to quantum systems includes reformulations of entropy and fluctuation relations in off-equilibrium dynamics.
Research leads
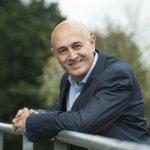
Professor Jim Al-Khalili
Professor
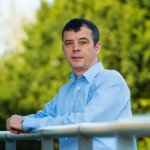
Professor Marian Florescu
Professor
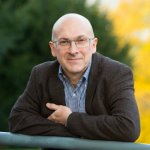
Dr Andrea Rocco
Associate Professor
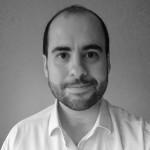
Dr Jesús Rubio Jiménez
Surrey Future Fellow
Overview
When a quantum system is no longer treated in isolation, but interacting with its surrounding environment, its quantum nature rapidly dissipates due an irreversible physical process called decoherence.
This process links with as yet not fully understood notions of quantum entropy and link with the emerging field of quantum thermodynamics, which is concerned with closing the gap between the small world of quantum mechanics and the large classical equilibrium world of thermodynamics by bringing together recent developments in both fields. It aims to build a new thermodynamic framework that goes beyond the conventional regime of validity of macroscopic thermodynamics to account for non-equilibrium dynamics at the quantum level.
Much of this work is funded by the John Templeton Foundation Project Life on the Edge: quantum thermodynamics, quantum biology and the Arrow of Time.
Research projects
Non-Markovian open quantum systems
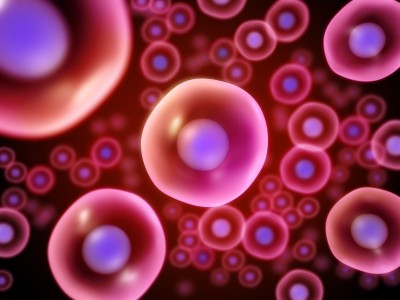
At Surrey, we have recently started the study of memory effects by generalising the Markovian approach of Caldeira and Leggett by adopting specific coarse-graining procedures.
We are further expanding this approach and consider the corresponding classical limit in terms of generalized Langevin and generalized Fokker-Planck equations, with the associated Fluctuation-Dissipation relations and entropy functions. We are also analysing extreme memory-reach environments, leading on the macroscopic scale to anomalous diffusion properties described by behaviours non-local in time, such as fractional macroscopic dynamics.
Research to understand the nature of open quantum systems inside living cells – how life has evolved the ability to use the environment within a cell to its advantage. Studying open quantum systems within biology provides an excellent testing ground for the most extreme way that quantum systems interact with their surroundings.
We are also interested in developing models of open quantum systems in and of themselves, with a view to better understanding decoherence in these systems. Such research has the potential to provide extremely valuable insights to the wider quantum field, for instance taking an understanding of the processes in biological systems to provide a template for quantum computing researchers to address problems in an artificial system.
Distinguishing between classical and quantum thermodynamics
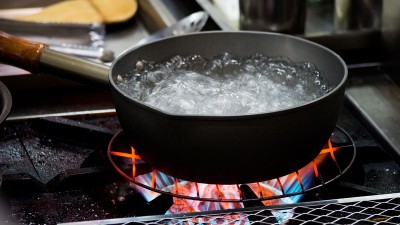
The derivation of thermodynamics from microscopic dynamics continues to be a challenging task. It is better understood both classically and quantum-mechanically for so-called memoryless systems, where it leads to equilibrium macroscopic dynamics and ordinary thermodynamical properties. However, when memory is present, deviations from standard thermodynamics are expected.
Exploring these deviations requires a novel framework in which the laws of thermodynamics are rederived from the microscopic interaction of a system of interest with its own environment, a so-called open system. This implies a reformulation of entropy functions and fluctuation-dissipation relations so as to characterize the resulting off-equilibrium dynamics at macroscopic scales and opens the way to a new understanding of the emergence of macroscopic irreversibility.
Research on the thermodynamic connection: using thermodynamics as a proxy of quantum-ness, in recognition that quantum thermodynamics is different from classical thermodynamics. Therefore, if we can make predictions in pathological situations in which classical thermodynamics fails to describe the system and we have to invoke quantum thermodynamics, then we have an argument to say the underlying dynamics must be quantum.
Non-Markovian quantum dynamics in structured photonic reservoirs
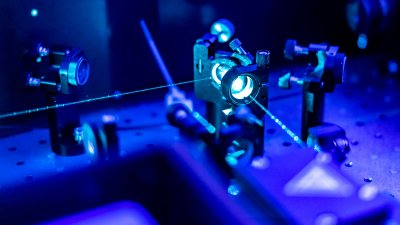
The ability to localize light and to engineer electromagnetic dispersion and local field enhancements in microstructured photonic systems facilitates enhanced optical nonlinearities, which is of great relevance for quantum optical information processing. The abrupt discontinuities in the engineered electromagnetic density of states of these materials give rise to non-Markovian evolution to the open quantum systems coupled to them dramatically enriching the landscape of the quantum phenomena observed.
Our team's interests lie in the exploration the dynamics of atomic ensembles in structured photonic reservoirs associated with photonic band-gap materials and photonic cavity systems and the ability to control the dynamics of quantum systems of interest thought a careful engineering of the surrounding electromagnetic environment.
We are currently exploring the correlation between the intrinsic non-Markovian effects associated with structured reservoirs and super-radiant behaviour, enhancements of energy transfer and reduction of decoherence, which prove essential in the fundamental understanding of the dynamics of open quantum systems and in the development of future quantum technologies.