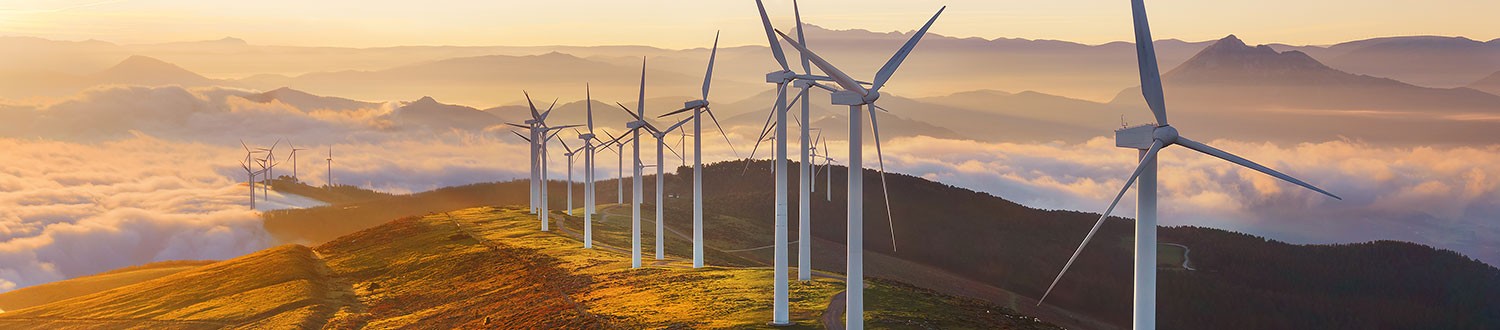
Centre for Infrastructure Systems Engineering
Our infrastructure systems engineering covers activities across a wide range of spatial and temporal scales aimed at sustainable infrastructure delivery and management.
Overview
Our research encompasses the behaviour of complex structural systems under cyclic, dynamic and extreme loads, the life-cycle characterisation and modelling of damage processes, and the performance management of individual assets and large infrastructure networks. We investigate the enormous potential of digital technologies, including sensing and machine learning algorithms, telecommunication engineering and data science on smart infrastructure and its social, environmental and economic impact on society. Advanced physical testing plays a key part in our research, with tests possible on a wide spectrum of specimens, from single fibres to full-scale bridge sub-assemblies in loading frames ranging from 1N to 1MN.
Our staff and research students bring together core competencies in materials, mechanics and structural engineering, with expertise in statistical methods, informatics and decision theory. We have established collaborations with a variety of industry partners, enabling us to analyse real data from critical infrastructure assets and to influence the development of industry guidelines on design, inspection and maintenance.
Research areas
- Asset integrity management of transport and water infrastructure networks
- Infrastructure monitoring and data analytics
- Innovative construction materials
- Hybrid and composite construction systems
- Modelling and testing of structures under static/dynamic/fatigue loading
- Risk and resilience of infrastructure assets and networks
- Robustness and progressive collapse of structural systems
- Spatial structures: form development and optimisation
- Urban systems growth modelling and nature-based solutions.
People
Academic staff
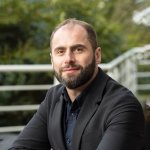
Dr Dan Bompa
Senior Lecturer | Structures and Materials Testing Lab Lead
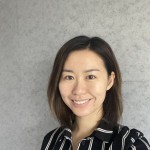
Dr Yidu Bu
Lecturer in Civil and Environmental Engineering
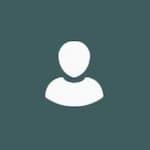
Professor Marios Chryssanthopoulos
Professor of Structural Systems
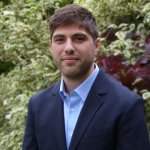
Dr Marco Funari
Lecturer in Civil and Environmental Engineering
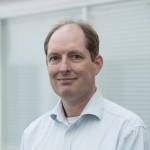
Dr Alex Hagen-Zanker
Associate Professor in Infrastructure Systems
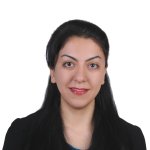
Dr Donya Hajializadeh
Associate Professor of Structural Engineering
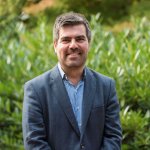
Dr Boulent Imam
Associate Professor (Reader) in Civil Engineering
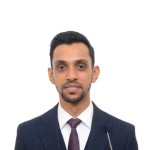
Sifan Muhamad Ibrahim
Lecturer in Structural Engineering
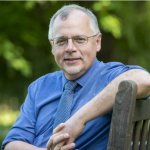
Dr Michael Mulheron
Reader in Construction Materials, Director of Learning and Teaching for Civil and Environmental Engineering
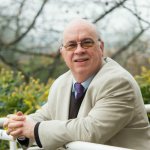
Professor Gerard Parke
Emeritus Professor of Structural Engineering
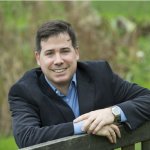
Dr Juan Sagaseta
Professor of Structural Robustness
Research staff
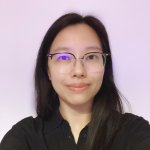
Dr Jingyan Yu
Research Fellow
Postgraduate research students
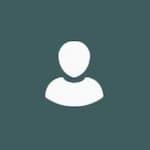
Dr Samuel Nkereuwem
Optimising structural elements containing hybrid fibre-reinforced concrete
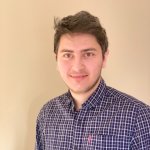
Chia Sadik
Postgraduate research student
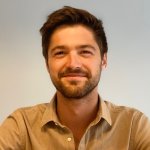
Pablo Vallhonrat Blanco
Robustness of bridge structures under extreme hydraulic actions
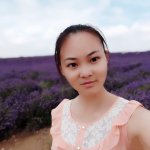
Chenmeng Zhang
Performance of one-part multi-binder alkali-activated materials and components