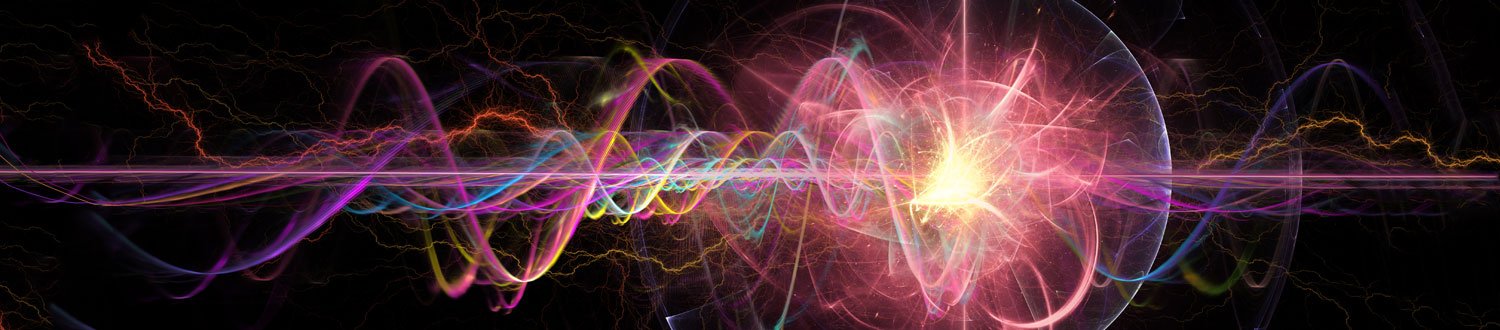
Research
Our group carries out research primarily to study quantum systems, both theoretically and experimentally, from the formal mathematical foundations of quantum mechanics to its applications in technology.
Selected publications
- T Guff and A Rocco, Time-reversal symmetry in open classical and quantum systems, arXiv:2311.08486
- L Buxton, MT Russo, J Al-Khalili, and A Rocco, Quantum Brownian motion in the Caldeira-Leggett model with a damped environment, arXiv:2303.09516
- YH Li, J Al-Khalili, and P Stevenson, A quantum simulation approach to implementing nuclear density functional theory via imaginary time evolution, arXiv:2308.15425
- L Slocombe, J Al-Khalili, and M Sacchi, Multiscale simulations reveal the role of PcrA helicase in protecting against proton transfer in DNA, ChemRxiv
- L Slocombe, M Winokan, J Al-Khalili, and M Sacchi, Quantum tunnelling effects in the Guanine-Thymine wobble misincorporation via tautomerisation, J Phys Chem Lett 14 (2023) 9
- B King, M Winokan, P Stevenson, J Al-Khalili, Louie Slocombe, and Marco Sacchi, Tautomerisation mechanisms in the Adenine-Thymine nucleobase pair during DNA strand separation, J Phys Chem B 127 (2023) 4220
- N Granchi, F Intonti, M Florescu, PD Garcia, M Gurioli, and G Arregui, Q-factor optimization of modes in ordered and disordered photonic systems using non-hermitian perturbation theory, ACS Photonics 10 (2023) 2808
- N Granchi, R Spalding, K Stokkereit, M Lodde, M Petruzzella, F Otten, R Sapienza, A Fiore, M Florescu, and F Intonti, High spatial resolution imaging of light localization in hyperuniform disordered patterns of circular air pores in a dielectric slab, Front Photon 4 (2023) 1199411
- A Burgess, M Florescu, and D Rouse, Strong coupling dynamics of driven quantum systems with permanent dipoles, AVS Quantum Sci 5 (2023) 031402
- A Burgess and M Florescu, Quantum memory effects in atomic ensembles coupled to photonic cavities, AVS Quantum Sci 5 (2023) 011402
- N Granchi, M Lodde, K Stokkereit, R Spalding, PJ van Veldhoven, R Sapienza, A Fiore, M Gurioli, M Florescu, and F Intonti, Near-field imaging of optical nanocavities in hyperuniform disordered materials, Phys Rev B 107 (2023) 064204
- SA Haver, E Ginossar, SE de Graaf, and E Grosfeld, Electromagnetic response of the surface states of a topological insulator nanowire embedded within a resonator, Commun Phys 6 (2023) 101
- NH Le, M Cykiert, and E Ginossar, Scalable and robust quantum computing on qubit arrays with fixed coupling, NPJ Quant Info 9 (2023)
- DC Brody, Quantum formalism for cognitive psychology, Sci Rep 13 (2023) 16104
- DC Brody and LP Hughston, Levy models for collapse of the wave function, J Phys A 56 (2023) 125303
- DC Brody and AJ Trewavas, Biological efficiency in processing information, Proc R Soc A 479 (2022) 0809
- S Lally, N Werren, J Al-Khalili, and A Rocco, Master equation for non-Markovian quantum Brownian motion: the emergence of lateral coherences, Phys Rev A 105 (2022) 012209
Selected PhD theses
- M Cykiert, Robust control of the transmon qubit for quantum information processing, supervisor: E Ginossar
- A Burgess, Non-Markovian dynamics of two-level systems in bosonic environments, supervisor: M Florescu
- E Lupo, Theory and design of quantum devices with superconducting circuits and topological states of matter, supervisors: E Ginossar and S Clowes
- MS Lally, Coherence dynamics in non-Markovian quantum Brownian motion, supervisors: J Al-Khalili and A Rocco
- L Buxton, Brownian motion in the Caldeira-Leggett model with a damped environment, supervisor: A Rocco
- L Slocombe, Quantum effects in DNA replication fidelity, supervisors: M Sacchi and J Al-Khalili
- R Spalding, Active hyperuniform disordered materials, supervisor: M Florescu