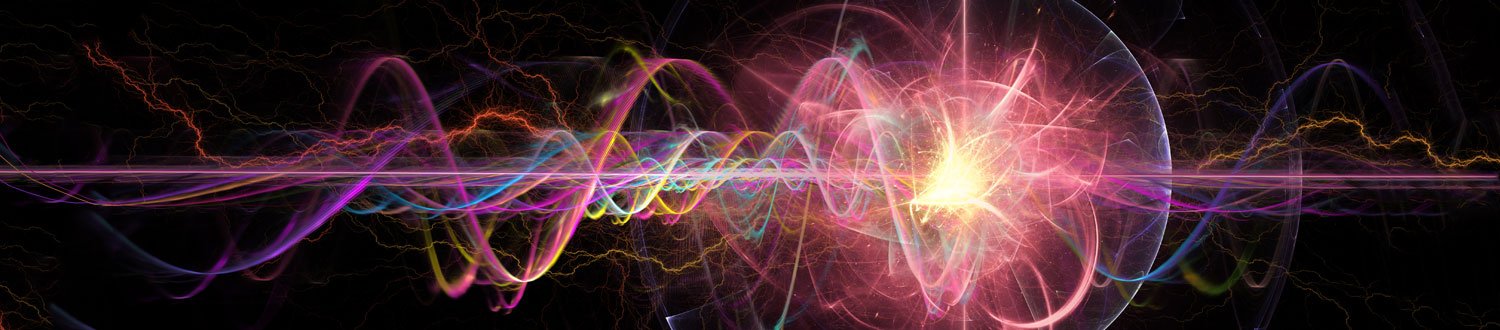
Quantum optics and photonics
Controlling optical properties of materials through hyperuniform disordered structure, New ways to control quantum dynamics in superconducting qubits, and novel non-linear optical effects.
Research leads
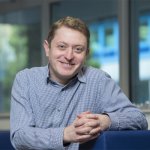
Professor Steven Clowes
Professor
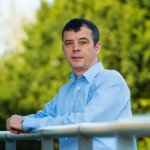
Professor Marian Florescu
Professor
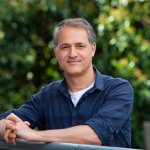
Dr Eran Ginossar
Associate Professor
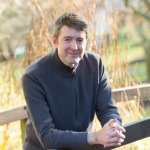
Professor Benedict Murdin
Professor
Overview
The use of light to communicate and process information is widely recognised as the technology that will drive innovations in the 21st century across a wide range of areas, from information technology, energy and sensing, to healthcare. So far, control of light flow has been achieved by carefully and periodically structured materials, which can bend light, slow it down and stop if for a short time, to allow for the processing steps to take place. Due to recent advances in theoretical, computational and nano-fabrication capabilities we are no longer restricted to well-defined periodic structures. Instead we can construct complex systems made of apparently random patterns, which when suitably designed, can lead to performances superior to those offered by conventional photonic systems.
Our team based in the Advanced Technology Institute is leading the development of hyperuniform and local self-uniform disordered nanophotonic materials, novel classes of photonic structures in which structural correlations and disorder are accurately controlled with the aim to control light flow, enhance light emission, and construct novel types of nanophotonic devices.
Quantum optical in hyperuniform disordered materials
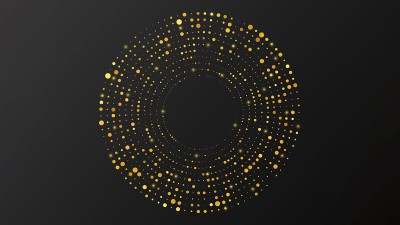
By exploiting the intrinsic statistical isotropy of the electromagnetic properties of the hyperuniform disordered materials, we are exploring new phenomena in optically active hyperuniform disordered systems. This isotropic scattering occurring over a finite range of frequencies facilitates a variety of photon transport regimes, ranging from ballistic-like to diffusive transport and even Anderson localisation.
Our recent work has exploited hyperuniform disordered designs of slab architectures, use of embedded quantum dots for feeding the hyperuniform resonances, and near-field hyperspectral imaging with sub-wavelength resolution to explore the transition from localisation to diffusive transport and to demonstrate the presence of both Anderson-localised modes and confined cavity modes with high-quality factors, with small footprint, intrinsically reproducible and resilient to fabrication-induced disorder, paving the way for a novel photonic platform for quantum applications.
Energy harvesting and thermal radiation management
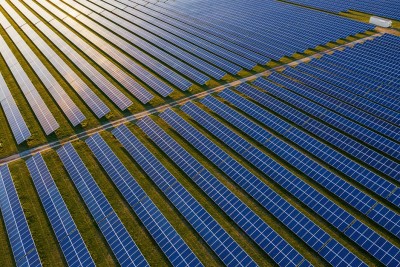
The ability of micro and nanostructured photonic materials to facilitate significant alteration of thermal radiation processes is receiving considerable attention due to both the scientific relevance and potential for technological applications.
Our research in this area is focused on the development of hyperuniform disordered nanophotonic metasurfaces, a novel type of structuring in which correlations and disorder are accurately controlled for achieving solar-thermal radiation conversion and management.
We have initiated a synergistic theoretical, computational and experimental approach to elucidate the fundamental correlation between the geometric and topological characteristics of the hyperuniform structures and their angle- and frequency-selective properties and to exploit the ability of nanostructured photonic materials to facilitate significant alteration of thermal radiation processes. This effort is supported by our recent demonstration of record-breaking efficiency in hyperuniformly textured thin-film silicon solar cells.
Structural colour and biomimetics
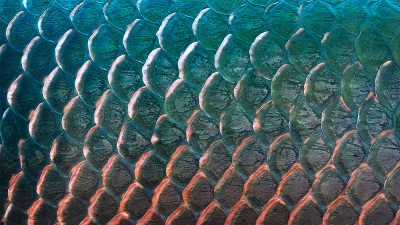
Studies of fish scales, insect coatings, mammal skin and bird feathers have revealed that membranes of living cells and intracellular organelles exhibit the capacity to self-organise into a variety of complex, nano to mesoscale structures similar to those in block-copolymers and other soft condensed matter systems. An accurate description of the colour-producing biological structures is essential for understanding their optical functionalities and can be directly exploited to the development and production of biomimetic devices that leverage comparable physical mechanisms.
Our research on photonic networks by their local self-uniformity has enabled a direct classification of advanced optical functionalities in materials as diverse as iridescent opals and the brilliantly white chaotic structure in Cyphochilus beetle scales, encompassing quasicrystals and glasses en route.
The local-self uniformity metric we have introduced not only sheds light on the regularity of fixed valency networks, but also establishes a direct connection between the structuring present in a myriad of photonic materials found in the natural world, and the advanced optical functionalities including structural colour. It can prove itself very useful in designing photonic structures with various functionalities, including spectral and directional control over the absorption of light and engineering structural colour.
THz non-linear optics
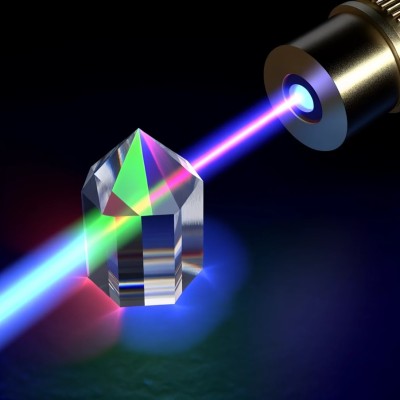
Our research has revealed that doped silicon exhibits an unprecedented non-linear optical coefficient, capable of photon manipulation at levels unseen in any other material.
This characteristic allows for the conversion of photons, akin to the process in green laser pointers where invisible near-infrared photons are transformed into visible light. Although requiring cryogenic temperatures, we are exploring the potential of this effect in specialised spectroscopy systems and other high-tech applications where cryogenic cooling is already utilised.