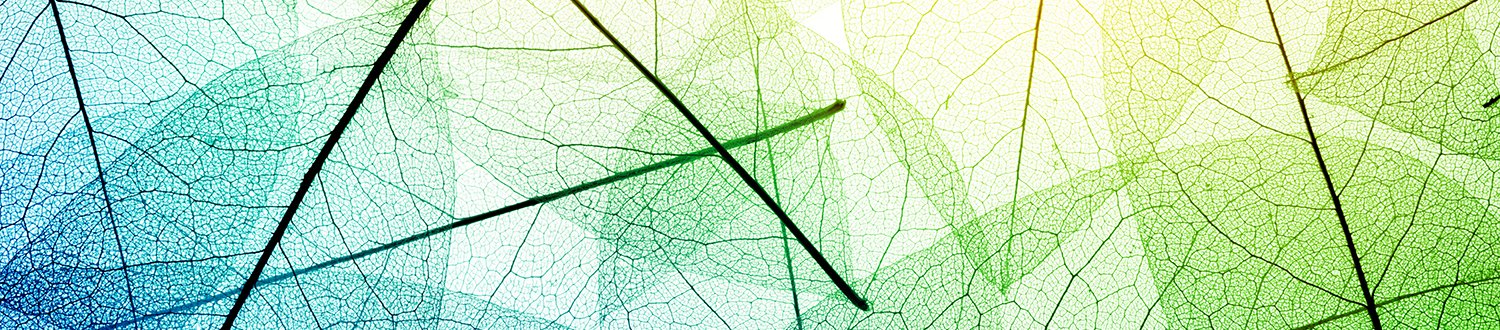
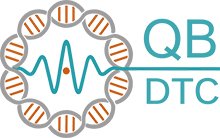
Quantum biophotonics
The advancement of optical methodologies applied to biological systems, such as ultrafast optical spectroscopy and single-molecule fluorescence spectroscopy, has provided new experimental observations suggesting that non-trivial quantum effects influence biological processes. In addition, recent observations in engineered fluorescent proteins amenable to quantum biological studies invite us to explore systematic approaches in studying biological quantum phenomena at the molecular level. These technical advances and scientific applications lead an exciting and emerging research area: Quantum Biophotonics.
About our work
The work in our Quantum Biophotonics Laboratory is focused on:
- Understanding how evolution has shaped biological systems that allow non-trivial quantum effects in biology
- Identification of new model systems for the study of quantum biology
- Development of new quantum-bio-inspired technologies.
Overview
Quantum mechanics has transformed our understanding of nature on an atomic scale and has been applied to many research areas and applications such as quantum information science and quantum computing in which experiments have been typically conducted in a carefully controlled laboratory environment. This is because quantum effects are fragile, i.e. random molecular interactions should obliterate quantum coherent molecular interactions in a warm and wet environment.
Evidence has been accumulated in recent years that non-trivial quantum effects may play a role in macromolecular interactions influencing biological processes. This is an exciting and emerging area of science that has recently gained huge attention. However, both developing experimental techniques and tractable biological systems amenable to investigating how nature maintains quantum effects in a warm, wet environment are urgently needed to resolve the many controversies in this field.
Quantum coherence effects within fluorescent protein (FP) at room temperature were thought to be impossible because close fluorophore proximity is limited by the FP ß-barrel structure, and the system-environment interactions at room temperature typically promote rapid vibrational decoherence [1]. Nonetheless, anomalous FPs behaviours have recently been observed in several studies suggesting that these fluorophores might have unique photophysical properties that preserve quantum effects under physiological conditions. For instance, it was observed that the dephasing time of green fluorescent protein (GFP) was measured to be about 1 ps [2], much longer than expected decoherence time of ~10 fs [1]. In addition, the generation of a polarization-entangled two photon state in enhanced GFP [3] and strong photon antibunching together with Davydov-splitting has been observed in homodimers of the YPF VenusA206 [4] have recently been reported. These results strongly support the hypothesis that FPs have evolved to maintain quantum effects under physiological conditions and might therefore have unique photophysical behaviours.
Thus, ultrafast optical spectroscopy of FPs can be used to extend our fundamental understanding of how nature can exploit quantum effects at ambient temperatures. They may also inspire new quantum-bio-inspired technologies such as the development of low-cost quantum computers or single-photon sources operating at room temperature.
Current projects
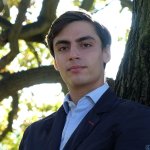
Room temperature coherent interactions between fluorescent proteins
Alejandro Sanchez-Pedreno Jimenez
Postgraduate researcher
Project description
Quantum mechanics has revolutionised our understanding of nature on how it works at the atomic and subatomic levels. Progress in quantum mechanics enabled us to develop practical electronic devices and opened a new field of studies amongst quantum information science and quantum computing. However, the extreme operating conditions such as cryogenic temperatures and vacuum environments required to exhibit quantum mechanical phenomena limit the large-scale applications. Similarly, the discovery of Green Fluorescent Protein (GFP) isolated from jellyfish Aequorea victoria initiated a revolution in the field of modern cell biology in which fluorescent protein has become a powerful and omnipresent tool for protein tagging and live-cell imaging. Fluorescent protein isolation from other organisms combined with mutagenic studies gave rise to diversify variants of fluorescent proteins. Yet, intrinsic photophysical properties have not been fully understood. Advancement of optical methodologies applied to the study of biological systems have allowed us to explore non-trivial quantum effects in biological processes.
This project aims to uncover the biophysical principles/mechanisms that enable to exhibit room temperature coherent molecular interactions between fluorescent proteins. Mutagenic studies will be applied to alter the structural integrity of fluorescent protein so that the mutants will be characterised using ultrafast optical spectroscopy.
Supervisors
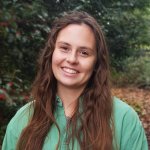
Highly efficient photon detection in the retina
Aimee Sweeney
Postgraduate researcher
Project description
Retina cells demonstrate the amazing capability of single-photon detection, pointing at the ability of biological photoreceptors to exhibit quantum mechanical properties at room temperature and normal atmospheric pressure, in a ‘noisy’ biological environment. In the human retina, photo transduction is an enormously efficient process, involving multi-wavelength photon absorption by different rod and cone cells and signal transfer through neurons towards the optical nerve. Several quantum processes have been suggested as mechanisms, contributing to the remarkable speed and efficiency of photoisomerization and subsequent signal transduction, including quantum coherence, superposition, and tunnelling in retina cells. Mimicking these processes with bio-compatible, synthetic organic molecular systems will provide pathways for a deeper understanding.
This project studies the use of naturally occurring chromophores isolated from the juice of different fruits and vegetables, for studies of their photoresponses in stimulated bio-environments when their peak absorption matches that of human eye rods and cones. A simplified model of the eye is being used to investigate is such chromophores can induce depolarisation effects to stimulate retina neuron depolarisation. The construction of a full colour response prosthetic retina ultra-flexible device will be attempted to investigate the feasibility of vision restoration. The study will also suggest strategies for the fabrication of single-photon bio-inspired molecular detectors that could help to increase the efficiency of solar cells.
Supervisors
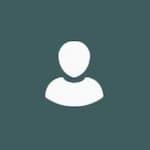
Developing bio-quantum technologies towards quantum enhanced biosensors
Anna Cusick
Postgraduate researcher
Project description
The advancement of observation techniques allows us to advance our understanding on how living systems exhibit quantum behaviour to achieve biological advantages that cannot be understood in the classical physics. Such quantum behaviour is thought to contribute to maintaining non-equilibrium state of biomolecular systems.
This project aims to explore the quantum aspects of fluorescent protein assemblies that prolong quantum behaviours under physiological conditions. We use quantum optics and femtosecond optical spectroscopy approaches, such as time-resolved fluorescence spectroscopy, photon antibunching and fluorescence lifetime correlation spectroscopy, to conduct this project. The knowledge created from this project may hold promise for next generation biological quantum technologies such as quantum-enhanced biosensors and even for our fundamental understanding of the life.
Supervisors
- Primary supervisor: Dr Youngchan Kim
- Co-supervisor(s): Professor Johnjoe McFadden.
Research team
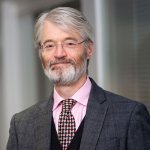
Professor Jeremy Allam
Professor of Ultrafast Optoelectronics
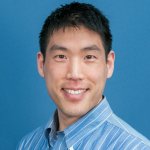
Dr Youngchan Kim
Lecturer in Quantum Biology, Director of the Quantum Biology Doctoral Training Centre (QB-DTC)
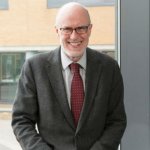
Professor Johnjoe McFadden
Professor of Molecular Genetics, Associate Dean (International)
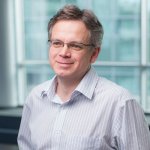
Dr Maxim Shkunov
Senior Lecturer
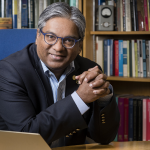
Professor Ravi Silva
Director, Advanced Technology Institute (ATI) Interim Director, Pan University Institute for Sustainability and Head of NanoElectronics Centre
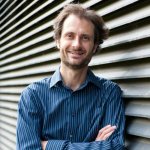
Dr Daniel Whelligan
Senior Lecturer in Organic/Medicinal Chemistry
References
- Gilmore, J. and R.H. McKenzie, Quantum dynamics of electronic excitations in biomolecular chromophores: role of the protein environment and solvent. The Journal of Physical Chemistry A, 2008. 112(11): p. 2162-2176.
- Cinelli, R.A., et al., Coherent dynamics of photoexcited green fluorescent proteins. Physical review letters, 2001. 86(15): p. 3439.
- Shi, S., P. Kumar, and K.F. Lee, Generation of photonic entanglement in green fluorescent proteins. Nature communications, 2017. 8(1): p. 1-7.
- Kim, Y., et al., VenusA206 Dimers Behave Coherently at Room Temperature. Biophysical journal, 2019. 116(10): p. 1918-1930.