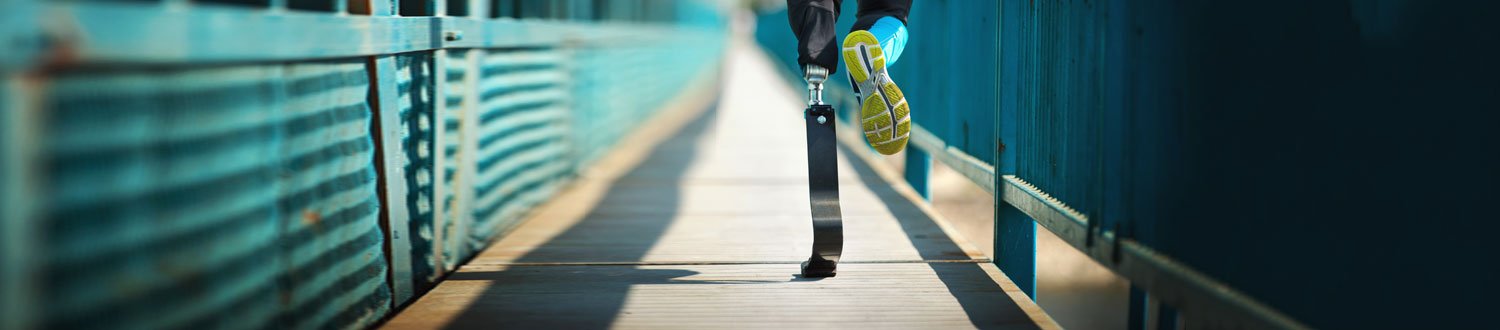
Research
Our goal is the application of engineering and technology to problems in medicine and biology, from the assessment, diagnosis and treatment of disease to a better understanding of the way the body works.
Collaboration
Over the years, our Centre has established links across the world, with world-leading companies such as Blatchfords, the Transport Research Laboratory and Smith and Nephew, hospitals across the south of England and extensive international research collaboration.
Research areas
We currently have thirteen research areas we are working on.
Bioelectronics
Whilst cells are usually considered solely as biochemical systems, they can also be considered as the sum of their interacting electrical aspects – membrane potentials, surface charges, resistances and capacitances. My group has focussed on the development of tools to exploit the electrical properties of cells.
We have built the world’s fastest cell separator (called EPACE), capable of separating a billion cells in thirty minutes. We have also built the first system (called 3DEP) capable of measuring many of these electrical properties – where conventional technology measures five cells a day, our technique measures 20,000 cells in ten seconds. We have also developed many applications for the technology, including cancer detection, and are now exploring the way in which these electrical properties interact – and how this influences cell behaviour in the body.
Dielectrophoresis and tissue engineering
In order to better understand biology from a new viewpoint, we have used electric fields and dielectrophoresis to determine the electric properties of cells.
These properties have been used to detect the presence of oral cancer in clinical samples (currently undergoing clinical trial), determine how stem cells will differentiate in order to select the right ones for therapeutic use, look at electrophysiological characteristics in circadian rhythms, as well as to study drug effects on various cells, and understand drug resistance in cancer.
We have applied the same technique to cell patterning, creating clusters of cells in order to better understand how cells work, grow and interact in 3D, particularly in understanding how this affect the way their electrophysiological properties change from 2D to 3D, and how drugs work.
Cerebrospinal fluid dynamics
The cerebrospinal fluid (CSF) is a clear fluid that bathes the brain and the spinal cord, protecting it from impact and vibration. CSF interacts with the cardiovascular system and is constantly moving in response to the arterial pulse. We are examining the movement of the CSF through experimental and theoretical models to see whether abnormal movement of the CSF relates to certain pathological conditions of the nervous system.
Computer models of the eye
Traumatic injury to the eye and the optic nerve, such as due to the impact of rapidly inflating airbags in automobiles, or a blunt object, can be simulated using computer models. These can be used both to minimise the risk of injury and to understand the exact mechanism through which various types of injury occur.
Osseointegration and orthopaedic implant design
We are part of an international collaboration to develop a new method of attachment. Titanium devices are implanted into the bone to which an artificial leg is attached, allowing the body weight to be supported through the skeleton. We also collaborate with clinicians on the design and performance evaluation of knee implants, and the tissue response to long-term implantation.
Functional electrical stimulation
When muscles and nerve pathways become damaged or have their function reduced as a result of disease, trauma or congenital condition, it is possible to restore some of the lost function by applying electrical stimulation to the remaining healthy tissue. Our work in functional electrical stimulation has allowed patients with such conditions as cerebral palsy, or ‘drop foot’ as a result of stroke, to improve their mobility.
Human movement and virtual reality
Understanding the way in which the human body moves is fundamental in understanding the basis of many diseases and in assessing patients with abnormal walking (gait), such as stroke patients and amputees.
Our human movement tools and expertise are now being applied to virtual reality and biofeedback techniques for gait re-education, for example, in post-stroke cases, and in areas of assessment of surgical skills, for example, by monitoring movement of the hands and fingers during clinical procedures.
Neural interfacing
The nervous system is the control network of the human body. We have developed implantable neuroprobes for communicating with the nervous system. These probes, much thinner than a human hair, contain electrodes that can record signals from individual nerve cells.
Our work covers many aspects of neural interfacing, including signal processing, probe construction and the assessment of the body’s reaction to the probe materials.
Tissue mechanics
Damage to tissue can have significant impact on health and wellbeing. Poor tissue health in the cardiovascular system, muscles of the pelvic floor and skin have very different but serious consequences. Understanding the causes and effects of tissue damage will help to improve the quality of life of individuals and provide guidance for new clinical interventions.
Tools that may traditionally be applied to heavy-duty mechanical structures are increasingly being translated to the delicate tissues found in biomedical engineering.
We are using novel computational simulations and experimental techniques to investigate the influence of microstructural tears on muscle contractions, the complex interaction of muscles necessary to maintain continence, and how skin behaviour can be linked to wearable sensors.
Shock wave therapy for the treatment of connective tissue conditions
Shock wave therapy (SWT) is a common term for therapeutic techniques that use high-amplitude transient pressure waves to treat a wide range of clinical conditions. While SWT was originally developed as a technique for breaking kidney stones, it is now being increasingly used for the management of a variety of musculoskeletal conditions such as the inflammation of tendons and ligaments in the foot.
At this point in time the understanding of the exact mechanisms through which pressure waves may work to promote healing in connective tissue is at the level of speculation. We have developed finite element models of a ballistic shock wave source and of the foot to examine the mechanical effects of SWT on the anatomical structures of the foot. The next step is to establish a link between the mechanical stimulus and the related therapeutically beneficial biological response.
Bioinspired design optimisation of biocomposites
Natural materials like human teeth and bones have complex and hierarchical architectures spanning a range of length scales, designed to achieve remarkable mechanical properties. However, it is still challenging to synthesize structures that can mimic the entire structural and mechanical characteristics of these natural materials.
Advanced manufacturing and processing techniques with fine microstructural control offer new possibilities for design and fabrication of biocomposites with tuneable structures and mechanical properties. This would provide biomimetic engineering solutions for dentistry, orthopaedics, and regenerative medicine. This interdisciplinary project investigates the relationship between nano- and micro- structures and micromechanics of natural and bioinspired biocomposites.
The objective is to elucidate the structural and mechanical key factors that affect the integrity, in particular the fracture behaviour in biocomposites as fabricated by innovative processing and manufacturing.
Biomedical signal processing
Analysis of the signals generated by the body with advanced signal processing techniques is fundamental in understanding the basis of many diseases. Our research focuses on the development of robust signal processing techniques for the analysis of different biomedical signals contaminated with noise.
These techniques are now being applied to the study of the electroencephalogram (EEG) in Alzheimer’s disease and mild cognitive impairment for early diagnosis of dementia, the development of diagnostic state and trait biomarkers in psychogenic non-epileptic seizures, the analysis of EEG in sleep and magnetoencephalograms for the characterisation of changes in the brain with healthy and pathological ageing, or to the study of electrocardiograms in cardiac autonomic neuropathy in diabetes.
Furthermore, we are developing algorithms for the analysis of multi-frequency biological rhythms (i.e. ultradian and circadian rhythms) for the extraction and classification of neurodegenerative biomarkers.
Applications of biomechanics and movement sensing
Many medical conditions can cause disruption of the way that an individual moves. Sometimes these effects are obvious, but subtle changes in the way an activity is performed can also indicate an underlying medical condition. Efficient measurement of human movement through wearable sensors can be useful in highlighting the need for further clinical assessment, identifying thresholds for interventions and in measuring an individual’s rehabilitation.
In the Human Movement Laboratory, we conduct experiments using optoelectronic systems, electromyography and accelerometers to analyse movement. These experiments are being applied in, for example, the identification of neurological damage, understanding of long-term effects of traumatic accidents and analysis of sports performance.
Study with us
Find a supervisor
Groups and centres
We work with the other groups and centres within the School of Mechanical Engineering Sciences.